Cation And Anion Held Together By Electrostatic Forces
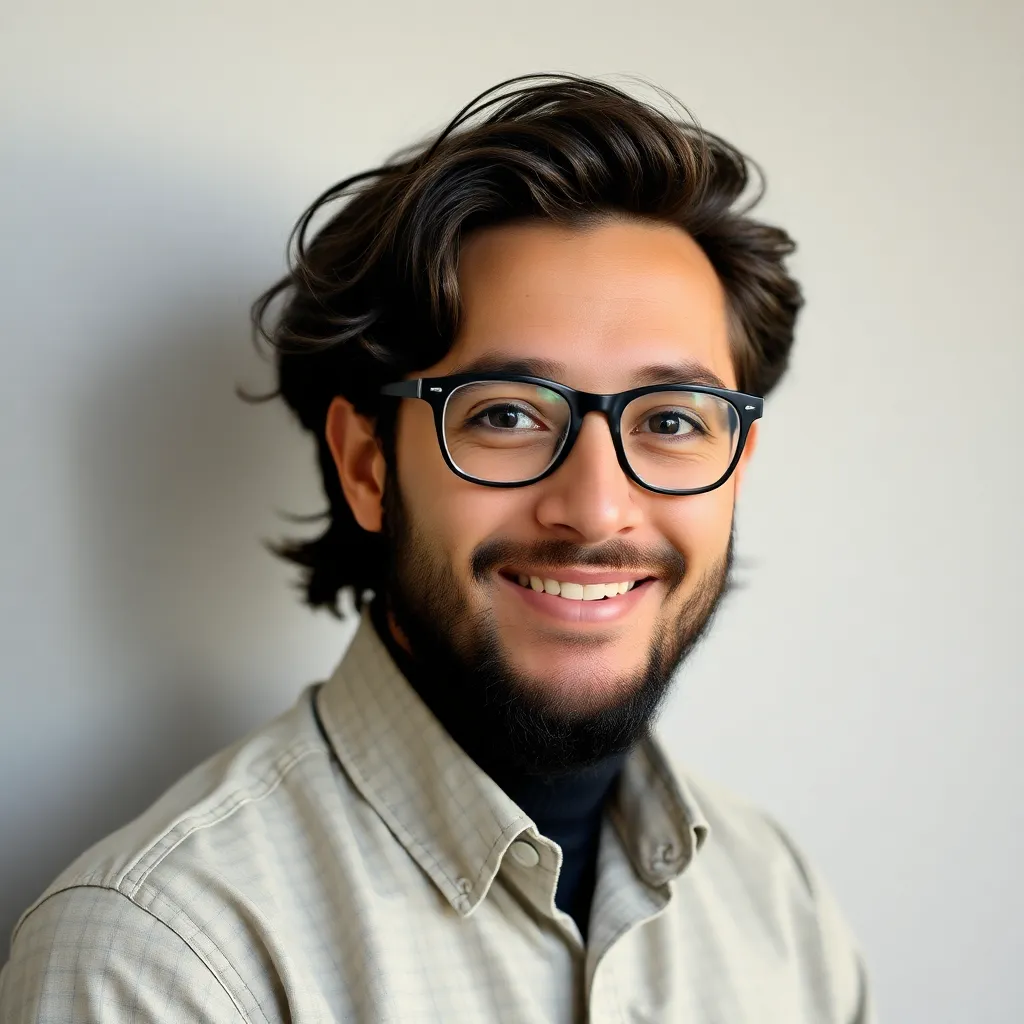
News Leon
Apr 01, 2025 · 7 min read
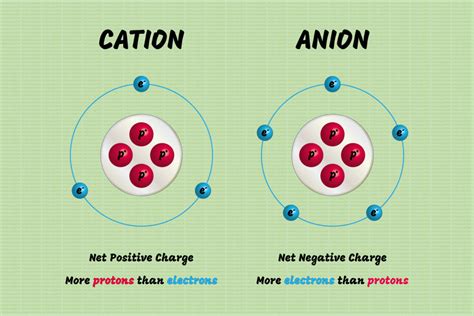
Table of Contents
Cations and Anions: A Deep Dive into Electrostatic Bonding
Ionic compounds, the bedrock of much of our material world, are held together by the powerful force of electrostatic attraction between oppositely charged ions. This fundamental interaction, a cornerstone of chemistry and physics, governs the properties of countless materials, from the salt we sprinkle on our food to the intricate structures of minerals deep within the Earth. Understanding the nature of this electrostatic bond, specifically the relationship between cations (positively charged ions) and anions (negatively charged ions), is crucial to grasping the behavior and applications of these materials.
The Genesis of Ionic Bonds: A Tale of Electron Transfer
The formation of an ionic bond hinges on the transfer of electrons from one atom to another. This transfer is driven by the inherent differences in electronegativity between the participating atoms. Electronegativity, a measure of an atom's ability to attract electrons towards itself in a chemical bond, plays a pivotal role in determining the likelihood of electron transfer and subsequent ionic bond formation.
Atoms with low electronegativity, typically metals located on the left side of the periodic table, readily lose electrons to achieve a stable electron configuration, often resembling the noble gas configuration of the previous period. This loss of electrons creates a positively charged ion, known as a cation. Conversely, atoms with high electronegativity, usually non-metals situated on the right side of the periodic table, tend to gain electrons to achieve a stable electron configuration, often mirroring that of the nearest noble gas. This gain of electrons results in a negatively charged ion, termed an anion.
Examples of Cation and Anion Formation
-
Sodium (Na) and Chlorine (Cl): Sodium, with a single electron in its outermost shell, readily loses this electron to become a +1 cation (Na⁺). Chlorine, with seven electrons in its outermost shell, readily gains an electron to become a -1 anion (Cl⁻). The electrostatic attraction between Na⁺ and Cl⁻ forms the iconic ionic compound, sodium chloride (NaCl), or common table salt.
-
Magnesium (Mg) and Oxygen (O): Magnesium, with two electrons in its outermost shell, loses these two electrons to become a +2 cation (Mg²⁺). Oxygen, with six electrons in its outermost shell, gains two electrons to become a -2 anion (O²⁻). This electron transfer leads to the formation of magnesium oxide (MgO), a crucial component in many industrial applications.
-
Aluminum (Al) and Nitrogen (N): Aluminum readily loses three electrons to form a +3 cation (Al³⁺), while nitrogen gains three electrons to form a -3 anion (N³⁻). This results in aluminum nitride (AlN), a material known for its high strength and thermal conductivity.
The Electrostatic Force: The Glue of Ionic Compounds
Once cations and anions are formed, the electrostatic force of attraction between their opposite charges holds them together. This force, described by Coulomb's Law, is directly proportional to the product of the charges and inversely proportional to the square of the distance between them:
F = k * (q₁ * q₂) / r²
Where:
- F is the electrostatic force
- k is Coulomb's constant
- q₁ and q₂ are the magnitudes of the charges of the ions
- r is the distance between the centers of the ions
This equation highlights the crucial role of charge magnitude and interionic distance in determining the strength of the electrostatic bond. Higher charges and shorter distances lead to stronger interactions.
The Influence of Ionic Radius and Charge Density
The size of the ions, their ionic radii, significantly impacts the strength of the electrostatic interaction. Smaller ions, with their concentrated charges, exhibit higher charge density, leading to stronger electrostatic forces. Consequently, ionic compounds formed by small, highly charged ions typically possess higher melting points and boiling points compared to those formed by larger ions with lower charges.
Crystal Lattice Structures: Order from Chaos
Ionic compounds do not exist as isolated ion pairs; instead, they organize into highly ordered, three-dimensional structures called crystal lattices. These lattices are arranged in a way that maximizes the electrostatic attractions between oppositely charged ions while minimizing the repulsions between like-charged ions. The specific crystal structure adopted depends on the relative sizes and charges of the cations and anions.
Several common crystal lattice structures include:
- Cubic Close-Packed (CCP): A structure with a high packing density, often found in compounds with similar cation and anion sizes.
- Body-Centered Cubic (BCC): Another common structure with a slightly lower packing density.
- Hexagonal Close-Packed (HCP): Similar to CCP in packing efficiency, but with a hexagonal arrangement of layers.
- Rock Salt (NaCl) Structure: A particularly common structure, characterized by a face-centered cubic arrangement of both cations and anions.
The crystal lattice structure is a fundamental determinant of the physical properties of the ionic compound, influencing its hardness, brittleness, and cleavage properties.
Properties of Ionic Compounds: A Reflection of Electrostatic Forces
The strong electrostatic forces holding ionic compounds together manifest in several distinctive properties:
-
High Melting and Boiling Points: The substantial energy required to overcome the strong electrostatic attractions results in high melting and boiling points.
-
Hardness and Brittleness: Ionic crystals are relatively hard due to the strong electrostatic forces. However, they are also brittle because dislocation of the ions can lead to repulsion between like charges, causing fracture.
-
Solubility in Polar Solvents: Ionic compounds often dissolve readily in polar solvents like water. The polar nature of water molecules allows them to effectively solvate the ions, reducing the electrostatic attractions between them and facilitating dissolution.
-
Electrical Conductivity: Ionic compounds are typically poor conductors of electricity in their solid state due to the fixed positions of the ions. However, they become good conductors when molten or dissolved in a polar solvent, as the ions become mobile and can carry electric charge.
Applications of Ionic Compounds: A Diverse Landscape
The versatility of ionic compounds is reflected in their extensive applications across various fields:
-
Table Salt (NaCl): Essential for human life and widely used as a seasoning and preservative.
-
Calcium Carbonate (CaCO₃): Used in construction materials, as an antacid, and in the production of cement.
-
Sodium Hydroxide (NaOH): A strong base used in various industrial processes, including soap making and paper production.
-
Potassium Chloride (KCl): Used in fertilizers and as a salt substitute.
-
Magnesium Oxide (MgO): Used as a refractory material, in medicine, and as an additive in various industrial processes.
-
Aluminum Oxide (Al₂O₃): A crucial component in abrasives, ceramics, and catalysts.
These are just a few examples; the vast array of ionic compounds and their applications highlights their significance in our daily lives and technological advancements.
Beyond the Basics: Advanced Concepts in Ionic Bonding
The simple picture of complete electron transfer painted above serves as a useful starting point, but the reality of ionic bonding is often more nuanced. Several factors can influence the degree of ionic character in a bond:
-
Polarization: The distortion of the electron cloud of an anion by a nearby cation. This effect reduces the purely ionic character of the bond and introduces some covalent character. The extent of polarization depends on the size and charge of the ions involved. Smaller, highly charged cations are more polarizing.
-
Covalent Character: In some ionic compounds, a significant degree of covalent character can exist. This arises from the sharing of electrons between the cation and anion, blurring the lines between purely ionic and purely covalent bonds.
Conclusion: The Enduring Importance of Electrostatic Forces
The interaction between cations and anions, held together by the powerful force of electrostatic attraction, forms the foundation of a vast and diverse class of materials. Understanding this fundamental interaction, including the nuances of electron transfer, crystal lattice structures, and the influence of factors like ionic size and polarization, is crucial for appreciating the properties and applications of ionic compounds. From the simple elegance of table salt to the complex functionalities of advanced materials, the electrostatic bond remains a central concept in chemistry and materials science, driving innovation and shaping our technological landscape. Continued research and exploration in this area will undoubtedly lead to further advancements and discoveries, further solidifying the importance of electrostatic interactions in the material world.
Latest Posts
Latest Posts
-
How Many Vertices Does Octagon Have
Apr 02, 2025
-
Reaction Of Ammonia And Sulfuric Acid
Apr 02, 2025
-
What Type Of Triangle Is Shown Below
Apr 02, 2025
-
Which Of The Following Pairs Are Mismatched
Apr 02, 2025
-
Why Displacement Is A Vector Quantity
Apr 02, 2025
Related Post
Thank you for visiting our website which covers about Cation And Anion Held Together By Electrostatic Forces . We hope the information provided has been useful to you. Feel free to contact us if you have any questions or need further assistance. See you next time and don't miss to bookmark.