3 Ways To Increase The Strength Of An Electromagnet
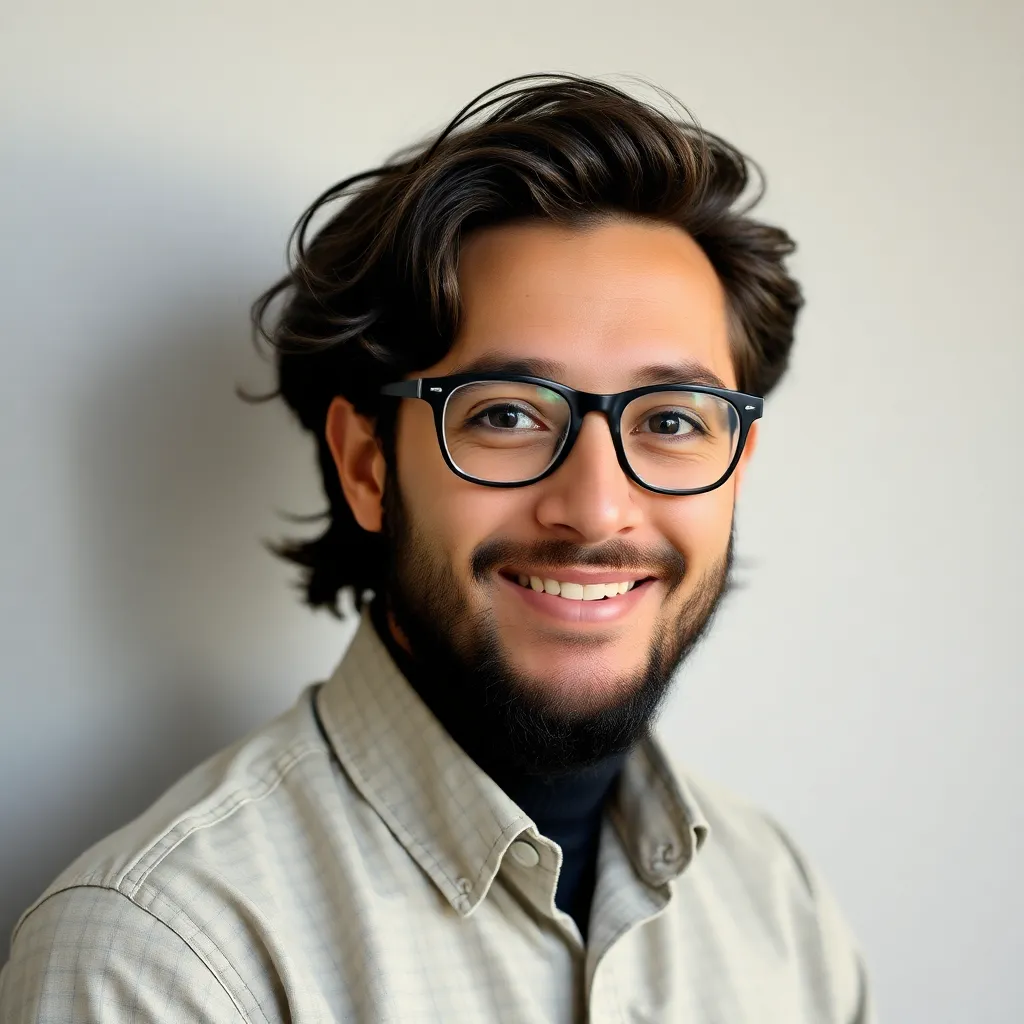
News Leon
Apr 07, 2025 · 6 min read
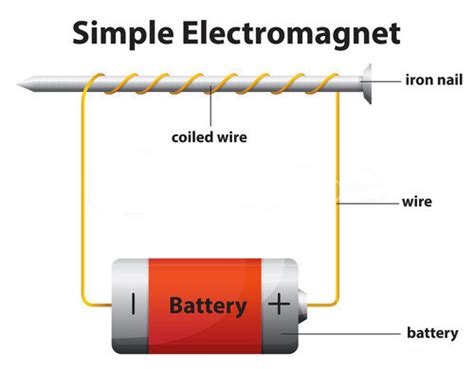
Table of Contents
3 Ways to Increase the Strength of an Electromagnet
Electromagnets, marvels of electromagnetic principles, find applications across diverse fields, from simple doorbells to sophisticated MRI machines. Their strength, the ability to generate a powerful magnetic field, is paramount to their functionality. Understanding how to amplify this strength is crucial for both practical applications and scientific exploration. This article delves into three primary methods for significantly increasing the strength of an electromagnet, providing a comprehensive understanding of the underlying physics and practical considerations involved.
1. Increasing the Number of Turns in the Coil
The foundation of an electromagnet lies in the current-carrying coil wrapped around a ferromagnetic core. The magnetic field's strength is directly proportional to the number of turns in this coil. Essentially, each turn contributes to the overall magnetic flux, leading to a cumulative strengthening effect.
The Physics Behind the Amplification
Faraday's law of induction is the cornerstone of this method. It states that the electromotive force (EMF) induced in a coil is proportional to the rate of change of magnetic flux through the coil. More turns mean a greater amount of magnetic flux linkage, resulting in a stronger magnetic field. This increase is linear; doubling the number of turns approximately doubles the magnetic field strength, assuming all other parameters remain constant.
Practical Considerations and Limitations
While increasing the number of turns seems straightforward, there are practical limitations:
-
Increased Resistance: Adding more turns to the coil increases its overall resistance. This leads to greater voltage drops across the coil, potentially requiring a higher voltage power supply to maintain the same current. A higher resistance also generates more heat, necessitating careful consideration of the coil's thermal capacity and insulation.
-
Space Constraints: Physical limitations might restrict the number of turns that can be wound onto a given core. The size and shape of the core, as well as the gauge of the wire, all play a crucial role in determining the maximum number of turns achievable.
-
Inductance: Increasing the number of turns also increases the coil's inductance. Higher inductance can affect the speed at which the electromagnet can switch on and off, potentially leading to slower response times in applications requiring rapid switching.
Optimizing for Maximum Strength
To maximize the strength while minimizing the drawbacks, consider these strategies:
-
Using Thicker Wire: Employing thicker wire with a lower resistance allows for a higher current at a given voltage, offsetting some of the resistance increase due to additional turns.
-
Efficient Coil Winding: Careful and even winding of the coil is vital to ensure uniform magnetic field distribution and maximize the magnetic flux linkage.
-
Optimal Core Material: Using a high-permeability core material significantly enhances the magnetic field strength by concentrating the magnetic flux lines. Materials like soft iron or mu-metal are commonly used for this purpose.
2. Increasing the Current Flow Through the Coil
The magnetic field produced by an electromagnet is directly proportional to the current flowing through its coil. Amplifying the current directly translates to an amplified magnetic field, a simple yet powerful method for enhancement.
Ampere's Law and Magnetic Field Strength
Ampere's law establishes the relationship between the magnetic field strength (H) and the current (I) circulating in a coil. The law states that the line integral of the magnetic field around a closed loop is proportional to the current enclosed by the loop. Increasing the current directly increases the magnetic field strength.
Practical Considerations and Challenges
Increasing the current, though seemingly straightforward, presents certain challenges:
-
Heat Dissipation: Higher current leads to increased Joule heating within the coil. The wire's temperature could rise significantly, potentially damaging the insulation and even melting the wire if adequate heat dissipation mechanisms aren't in place. This often necessitates the use of cooling systems, such as fans or water cooling, particularly for high-current applications.
-
Power Supply Limitations: Achieving higher currents requires a power supply capable of delivering the necessary voltage and current. The power supply must be sized appropriately to avoid overloading and potential damage.
-
Saturation of the Core: Increasing the current beyond a certain point may lead to saturation of the ferromagnetic core. Once saturated, the core can no longer effectively concentrate the magnetic flux, and further increases in current will yield diminishing returns in magnetic field strength.
Optimizing for Current Amplification
To effectively increase the current without compromising the system, consider:
-
Heat Sinks: Implementing heat sinks helps to dissipate the heat generated by the increased current, preventing overheating and ensuring safe operation.
-
Higher Gauge Wire: Using thicker wire (lower gauge) can handle higher currents without excessive heating due to its lower resistance.
-
Pulse Current Techniques: Applying pulsed currents, rather than continuous currents, can allow for higher peak currents without the continuous heat build-up associated with constant current flow.
3. Employing a Core Material with Higher Permeability
The core material's permeability plays a vital role in determining the electromagnet's overall strength. Permeability is a measure of how easily a material can be magnetized. A higher permeability material will concentrate the magnetic flux more effectively, resulting in a stronger magnetic field for the same amount of current and turns.
Permeability and Magnetic Flux Density
The magnetic flux density (B), a measure of the magnetic field's strength, is related to the magnetic field intensity (H) and the material's permeability (μ) through the equation B = μH. A higher permeability (μ) leads to a higher flux density (B) for a given magnetic field intensity (H).
Choosing the Right Core Material
Several materials exhibit high permeability and are commonly used in electromagnets:
-
Soft Iron: A readily available and relatively inexpensive material with good permeability, suitable for many general-purpose applications.
-
Mu-Metal: A nickel-iron alloy with exceptionally high permeability, often used in applications requiring highly sensitive magnetic fields, such as shielding against external magnetic interference.
-
Ferrite: Ceramic materials with high permeability, often used in high-frequency applications due to their lower eddy current losses.
Practical Considerations and Material Selection
The choice of core material depends heavily on the specific application. Factors to consider include:
-
Cost: Different core materials have varying costs, with more specialized high-permeability materials being more expensive.
-
Saturation: Even high-permeability materials can saturate at high magnetic field intensities. Choosing a material with a saturation point appropriate for the expected operating conditions is vital.
-
Hysteresis: Hysteresis refers to the energy lost during magnetization and demagnetization cycles. Materials with low hysteresis are preferred for applications requiring frequent switching or where energy efficiency is paramount.
-
Eddy Currents: Eddy currents are induced currents within the core that oppose the changing magnetic field, leading to energy loss and heating. Materials with high electrical resistivity, such as ferrites, minimize eddy current losses.
Conclusion: A Synergistic Approach
While each method independently contributes to increasing electromagnet strength, combining these techniques often yields the most significant results. For instance, using a high-permeability core material in conjunction with a coil having a large number of turns and carrying a high current creates a powerful electromagnet far exceeding the capabilities of any single method alone. The optimal configuration will depend on the specific application's constraints, such as size, cost, power availability, and required field strength. Careful consideration of these factors, coupled with a thorough understanding of the underlying electromagnetic principles, enables the design and construction of potent and efficient electromagnets for various applications. The key lies in achieving a synergistic effect, where the advantages of each method are maximized, and their limitations are mitigated. This balanced approach ensures that the resulting electromagnet possesses the desired strength, efficiency, and longevity.
Latest Posts
Latest Posts
-
What Organelle Is Considered A Factory
Apr 07, 2025
-
The Tendency Of An Atom To Attract Electrons
Apr 07, 2025
-
Determine The Potential Difference Va Vb Shown In The Circuit Below
Apr 07, 2025
-
Which Word Is An Antonym Of Gaudy
Apr 07, 2025
-
0 67 As A Fraction In Simplest Form
Apr 07, 2025
Related Post
Thank you for visiting our website which covers about 3 Ways To Increase The Strength Of An Electromagnet . We hope the information provided has been useful to you. Feel free to contact us if you have any questions or need further assistance. See you next time and don't miss to bookmark.