The Tendency Of An Atom To Attract Electrons
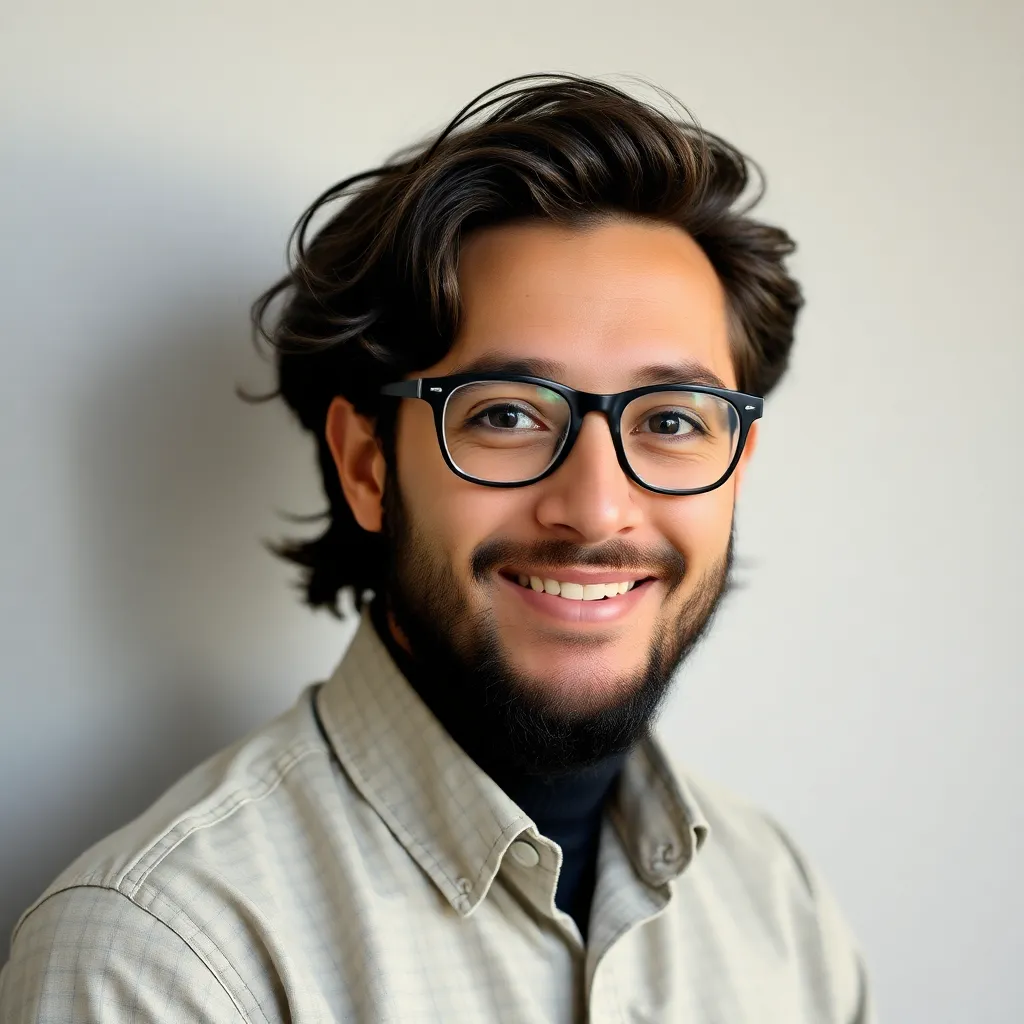
News Leon
Apr 07, 2025 · 6 min read
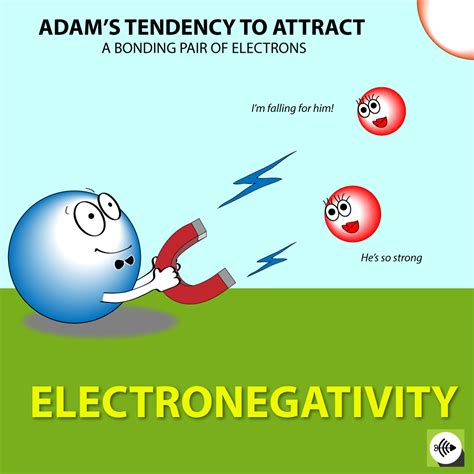
Table of Contents
The Tendency of an Atom to Attract Electrons: Electronegativity and its Chemical Consequences
The tendency of an atom to attract electrons towards itself when it is part of a chemical bond is a fundamental concept in chemistry known as electronegativity. Understanding electronegativity is crucial for predicting the behavior of atoms in molecules and explaining a vast range of chemical phenomena, from the formation of ionic and covalent bonds to the properties of acids and bases. This article delves into the intricacies of electronegativity, exploring its origins, trends in the periodic table, its influence on bond polarity and molecular geometry, and its crucial role in dictating chemical reactivity.
What is Electronegativity?
Electronegativity isn't a directly measurable quantity like mass or charge. Instead, it's a relative measure, comparing the ability of an atom within a molecule to attract shared electrons in a covalent bond. The greater an atom's electronegativity, the more strongly it pulls electrons towards itself. This pull influences the distribution of electron density within the molecule, leading to variations in bond character and overall molecular properties.
Factors Influencing Electronegativity
Several factors contribute to an atom's electronegativity:
-
Nuclear Charge: A higher positive charge in the nucleus exerts a stronger attractive force on electrons, increasing electronegativity. As you move across a period in the periodic table, the nuclear charge increases, leading to a general increase in electronegativity.
-
Atomic Radius: A smaller atomic radius means the electrons are closer to the nucleus, experiencing a stronger attractive force. Electronegativity generally decreases down a group in the periodic table because atomic radius increases.
-
Shielding Effect: Inner electrons shield outer electrons from the full positive charge of the nucleus. This shielding effect reduces the effective nuclear charge experienced by the valence electrons. The increased shielding effect down a group diminishes the electronegativity.
Trends in Electronegativity Across the Periodic Table
Electronegativity values are not absolute; they are relative to a standard. The most commonly used scale is the Pauling scale, where fluorine (F) is assigned the highest electronegativity value of 4.0. Observing the periodic table reveals clear trends:
-
Across a Period (Left to Right): Electronegativity generally increases from left to right. This is primarily due to the increasing nuclear charge, which outweighs the increase in shielding.
-
Down a Group (Top to Bottom): Electronegativity generally decreases from top to bottom. This is because the increasing atomic radius and shielding effect reduce the attractive force of the nucleus on the valence electrons.
The Most and Least Electronegative Elements
Fluorine (F) is the most electronegative element, consistently attracting electrons with the greatest force. At the other end of the spectrum, elements like francium (Fr) and cesium (Cs) exhibit the lowest electronegativities. Their large atomic radii and strong shielding effects significantly weaken the nucleus's pull on valence electrons.
Electronegativity and Bond Polarity
The difference in electronegativity between two bonded atoms directly impacts the nature of the bond they form.
-
Nonpolar Covalent Bonds: When two atoms with similar electronegativities bond, they share electrons relatively equally. The electron density is evenly distributed, resulting in a nonpolar covalent bond. Examples include bonds between identical atoms (e.g., H-H, Cl-Cl).
-
Polar Covalent Bonds: When two atoms with different electronegativities bond, the more electronegative atom attracts the shared electrons more strongly. This creates an uneven distribution of electron density, leading to a polar covalent bond. One end of the bond carries a partial negative charge (δ-), and the other end carries a partial positive charge (δ+). Water (H₂O) is a classic example, with the oxygen atom carrying a partial negative charge and the hydrogen atoms carrying partial positive charges.
-
Ionic Bonds: When the electronegativity difference between two atoms is very large, the more electronegative atom essentially steals an electron from the less electronegative atom. This results in the formation of ions: a positively charged cation and a negatively charged anion. The electrostatic attraction between these oppositely charged ions forms an ionic bond. Sodium chloride (NaCl) is a prime example, with sodium (Na) losing an electron to become a cation and chlorine (Cl) gaining an electron to become an anion.
Electronegativity and Molecular Geometry
The distribution of electron density, influenced by electronegativity, significantly affects a molecule's geometry. In polar molecules, the uneven distribution of charge creates a dipole moment, a vector quantity indicating the direction and magnitude of the molecule's polarity. This dipole moment influences intermolecular forces, affecting properties like boiling point, melting point, and solubility.
Influence on Intermolecular Forces
Polar molecules exhibit stronger intermolecular forces (like dipole-dipole interactions and hydrogen bonding) compared to nonpolar molecules (which primarily experience weaker London dispersion forces). These stronger intermolecular forces result in higher boiling and melting points. For instance, water's high boiling point is a direct consequence of its strong hydrogen bonds, which are facilitated by the polar nature of the O-H bonds.
Electronegativity and Chemical Reactivity
Electronegativity plays a critical role in determining the chemical reactivity of elements and compounds. Highly electronegative atoms tend to gain electrons readily, making them strong oxidizing agents. Conversely, atoms with low electronegativity readily lose electrons, acting as strong reducing agents.
Predicting Reaction Outcomes
By considering the electronegativity differences between reactants, we can often predict the outcome of chemical reactions. For example, the reaction between a highly electronegative element like fluorine and a low electronegative element like sodium is highly favorable because fluorine strongly attracts electrons from sodium, leading to the formation of an ionic compound.
Applications of Electronegativity
The concept of electronegativity is not confined to theoretical discussions. It has practical applications in various fields:
-
Drug Design: Understanding electronegativity helps in designing drugs with specific properties. The polarity of drug molecules, influenced by electronegativity, affects their absorption, distribution, metabolism, and excretion (ADME) properties.
-
Material Science: The electronegativity of constituent atoms is critical in predicting the properties of materials. It influences the type of bonding, affecting the material's strength, conductivity, and other physical characteristics.
-
Environmental Chemistry: Understanding electronegativity is vital for studying environmental processes, like the movement of pollutants in soil and water. The polarity of molecules influences their solubility and mobility in the environment.
Advanced Concepts and Related Topics
While the fundamental concepts of electronegativity are relatively straightforward, there are more sophisticated aspects to consider:
-
Charge Distribution and Resonance: In molecules with resonance structures, the actual charge distribution is a weighted average of the contributing structures. Electronegativity plays a role in determining the relative contribution of each resonance structure.
-
Mulliken Electronegativity: This scale provides a different perspective on electronegativity, relating it to ionization energy and electron affinity. It offers a more quantitative approach compared to the Pauling scale.
-
Electronegativity and Acid-Base Chemistry: The electronegativity of atoms in a molecule can influence its acidity or basicity. More electronegative atoms can stabilize negative charges, making a molecule more acidic.
-
Electronegativity and Catalysis: The electronegativity of catalyst atoms significantly impacts their ability to facilitate chemical reactions.
Conclusion
The tendency of an atom to attract electrons, its electronegativity, is a fundamental concept that underpins much of our understanding of chemical bonding, molecular structure, and reactivity. From predicting the polarity of bonds to understanding the behavior of molecules in various environments, electronegativity provides a powerful framework for interpreting and predicting chemical phenomena. Its importance extends far beyond the classroom, influencing fields like drug design, materials science, and environmental chemistry. A deep understanding of electronegativity is therefore essential for anyone seeking a comprehensive grasp of the chemical world.
Latest Posts
Latest Posts
-
Which Of The Following Can Serve As A Nucleophile
Apr 08, 2025
-
Oxidation Number Of S In So3
Apr 08, 2025
-
Replication Is Called Semi Conservative Because
Apr 08, 2025
-
Oxidation Number Of C In C2o4 2
Apr 08, 2025
-
Examples Of Right Angles In Real Life
Apr 08, 2025
Related Post
Thank you for visiting our website which covers about The Tendency Of An Atom To Attract Electrons . We hope the information provided has been useful to you. Feel free to contact us if you have any questions or need further assistance. See you next time and don't miss to bookmark.