Unsymmetrical Ethers Can Be Made By The Williamson Synthesis
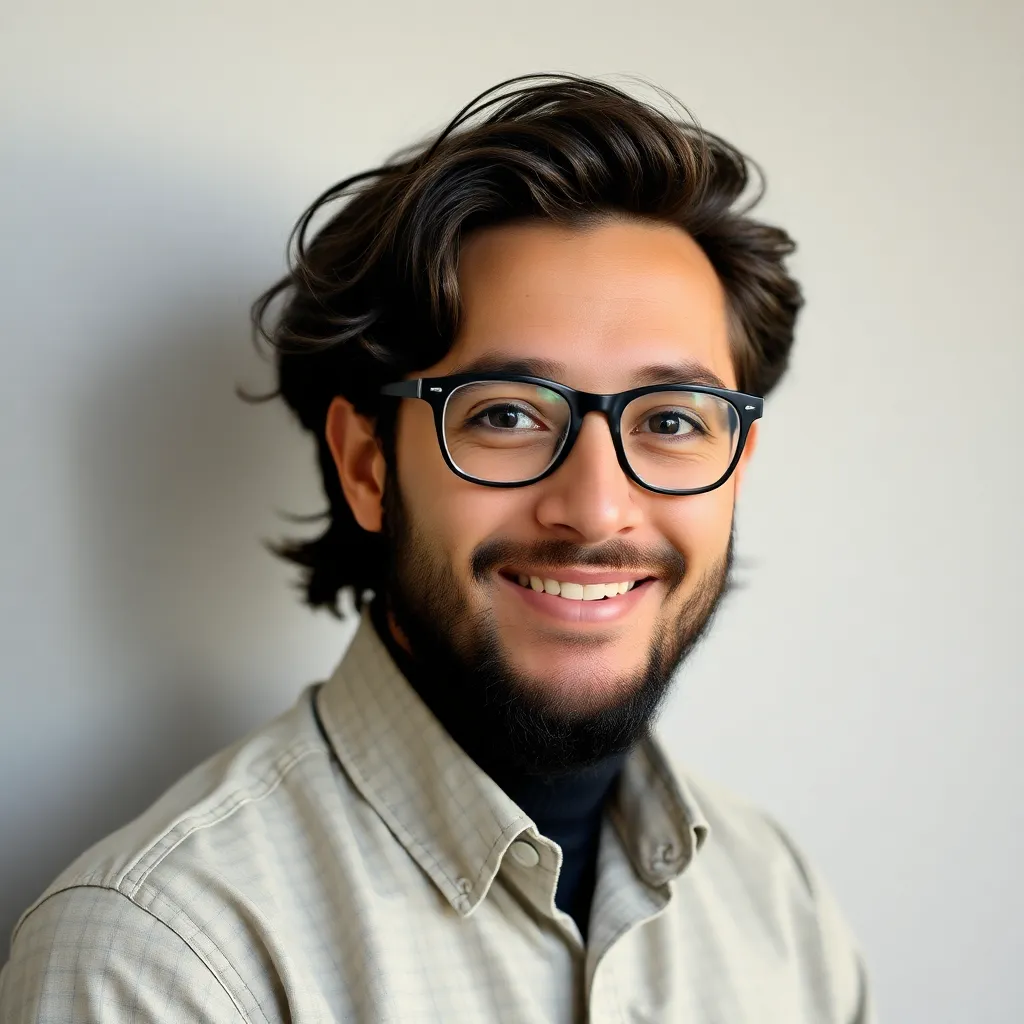
News Leon
Apr 05, 2025 · 5 min read
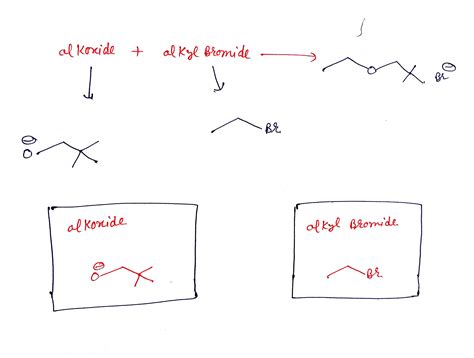
Table of Contents
Unsymmetrical Ethers: Williamson Synthesis and Beyond
The Williamson ether synthesis is a cornerstone of organic chemistry, providing a reliable method for the preparation of ethers. While it excels at creating symmetrical ethers, its application extends to the synthesis of unsymmetrical ethers as well, albeit with considerations for reaction selectivity and potential side reactions. This comprehensive article delves deep into the Williamson synthesis's role in crafting unsymmetrical ethers, exploring its mechanism, limitations, and modifications to overcome challenges. We'll also briefly discuss alternative methods for synthesizing this important class of organic compounds.
Understanding Unsymmetrical Ethers
Unsymmetrical ethers are characterized by having two different alkyl or aryl groups attached to the oxygen atom. Their structural diversity makes them valuable building blocks in various organic syntheses and finds applications in diverse fields, including pharmaceuticals, agrochemicals, and materials science. Examples include anisole (methoxybenzene), a common solvent and precursor in the perfume industry, and many pharmaceutical intermediates containing ether linkages. Synthesizing these compounds efficiently and selectively is crucial.
The Williamson Ether Synthesis: A Foundation for Ether Preparation
The Williamson ether synthesis is an SN2 reaction involving the reaction of an alkoxide ion with a primary alkyl halide or tosylate. The alkoxide acts as a nucleophile, attacking the electrophilic carbon atom of the alkyl halide, displacing the halide ion and forming the ether linkage. This reaction is particularly effective for creating symmetrical ethers where both alkyl groups are identical.
The Mechanism of Williamson Ether Synthesis
The reaction proceeds through a concerted SN2 mechanism:
-
Formation of the Alkoxide Ion: A strong base, such as sodium hydride (NaH) or potassium tert-butoxide (t-BuOK), deprotonates an alcohol, generating the corresponding alkoxide ion. This alkoxide ion is a crucial nucleophile in the subsequent step.
-
Nucleophilic Attack: The alkoxide ion, possessing a negatively charged oxygen atom, attacks the electrophilic carbon atom of the alkyl halide or tosylate. This attack occurs from the backside of the carbon atom, leading to inversion of configuration at that center (if a chiral center is involved).
-
Displacement and Ether Formation: The halide or tosylate leaving group departs, and a new C-O bond is formed, yielding the ether product.
Synthesizing Unsymmetrical Ethers via Williamson Synthesis
The key to successfully synthesizing unsymmetrical ethers using the Williamson ether synthesis lies in strategic choice of the alkyl halide and alkoxide. The crucial factor is minimizing competing side reactions, especially elimination reactions.
Choosing the Right Reactants: A Strategic Approach
The success of Williamson synthesis for unsymmetrical ether preparation hinges on selecting the appropriate alkoxide and alkyl halide. Generally, it's advisable to use:
-
A strong nucleophile (alkoxide): The alkoxide ion should be a relatively strong nucleophile to ensure efficient attack on the alkyl halide. Sterically hindered alkoxides can be less reactive.
-
A good leaving group (halide/tosylate): The alkyl halide or tosylate should have a good leaving group (I⁻ > Br⁻ > Cl⁻ > OTs⁻). This facilitates the displacement step in the SN2 mechanism.
-
A primary or methyl halide/tosylate: Primary alkyl halides or methyl halides are preferred. Secondary alkyl halides are less suitable because they are prone to elimination reactions competing with the SN2 pathway. Tertiary alkyl halides generally do not participate in SN2 reactions effectively.
Example: To synthesize ethyl methyl ether, one would react sodium methoxide (CH₃ONa) with iodoethane (CH₃CH₂I). Sodium methoxide provides the methoxide nucleophile, while iodoethane provides the ethyl group and a good leaving group (iodide).
Avoiding Elimination Reactions: A Critical Consideration
Elimination reactions are significant side reactions in Williamson synthesis, particularly when secondary or tertiary alkyl halides are used. These reactions compete with the SN2 pathway, reducing the yield of the desired ether. Elimination leads to the formation of alkenes. To minimize elimination:
-
Use primary or methyl halides: As stated earlier, primary and methyl halides greatly minimize the chances of elimination reactions.
-
Use milder reaction conditions: Employing lower temperatures can slow down elimination reactions relative to the SN2 substitution pathway.
-
Choose a suitable base: The base strength needs careful consideration. While a strong base is needed for alkoxide formation, an excessively strong base may promote elimination.
Alternative Williamson Strategies for Unsymmetrical Ether Synthesis
Sometimes, direct Williamson synthesis might not be optimal. Here are alternative approaches:
-
Reverse the order of reactants: Instead of using a primary halide and an alkoxide, consider using a primary alcohol and an alkyl halide. This changes the reactivity landscape.
-
Protecting group strategies: For more complex substrates, protecting groups might be necessary to prevent unwanted reactions at other functional groups. Careful planning and removal of the protecting group are crucial.
Limitations of Williamson Synthesis for Unsymmetrical Ethers
While versatile, the Williamson synthesis has limitations:
-
Steric hindrance: Bulky alkyl groups can hinder the SN2 reaction, reducing yield.
-
Competing reactions: Elimination reactions are a major concern, especially with secondary or tertiary halides.
-
Rearrangements: In certain cases, carbocation rearrangements can occur, leading to unexpected products.
Alternative Methods for Unsymmetrical Ether Synthesis
Besides the Williamson synthesis, other methods exist for synthesizing unsymmetrical ethers:
-
Acid-catalyzed dehydration of alcohols: This method involves reacting two different alcohols in the presence of a strong acid catalyst. However, it's limited by the need for relatively reactive alcohols and the possibility of forming symmetrical ethers as byproducts.
-
Alkoxymercuration-demercuration: This method utilizes mercuric acetate and an alcohol to add an alkoxy group to an alkene. Subsequent reduction with sodium borohydride replaces mercury with hydrogen.
-
Ullmann condensation: This copper-catalyzed reaction couples aryl halides with phenols or other aromatic alcohols to form aryl ethers.
Conclusion: Optimizing Williamson Synthesis for Unsymmetrical Ethers
The Williamson ether synthesis remains a highly valuable and widely used method for preparing both symmetrical and unsymmetrical ethers. While it presents some limitations, careful consideration of reactant choice, reaction conditions, and potential side reactions allows for successful synthesis of unsymmetrical ethers in many cases. Understanding the mechanism, choosing appropriate reactants, and taking into account the potential for competing reactions are essential for maximizing yield and obtaining the desired product. The availability of alternative methods offers further flexibility in the design and execution of synthetic strategies when the Williamson approach is less suitable. The versatility and robustness of the Williamson synthesis continue to make it an indispensable tool in the organic chemist’s arsenal.
Latest Posts
Latest Posts
-
The Cell Wall In Bacteria Is Primarily Composed Of
Apr 05, 2025
-
What Is 2 3 Repeating As A Fraction
Apr 05, 2025
-
Constant Returns To Scale Production Function
Apr 05, 2025
-
What Is The Index Of Refraction For Crown Glass
Apr 05, 2025
-
Which Is Greater 536 Cm Or 53 6 Dm
Apr 05, 2025
Related Post
Thank you for visiting our website which covers about Unsymmetrical Ethers Can Be Made By The Williamson Synthesis . We hope the information provided has been useful to you. Feel free to contact us if you have any questions or need further assistance. See you next time and don't miss to bookmark.