Three Parts Of An Atp Molecule
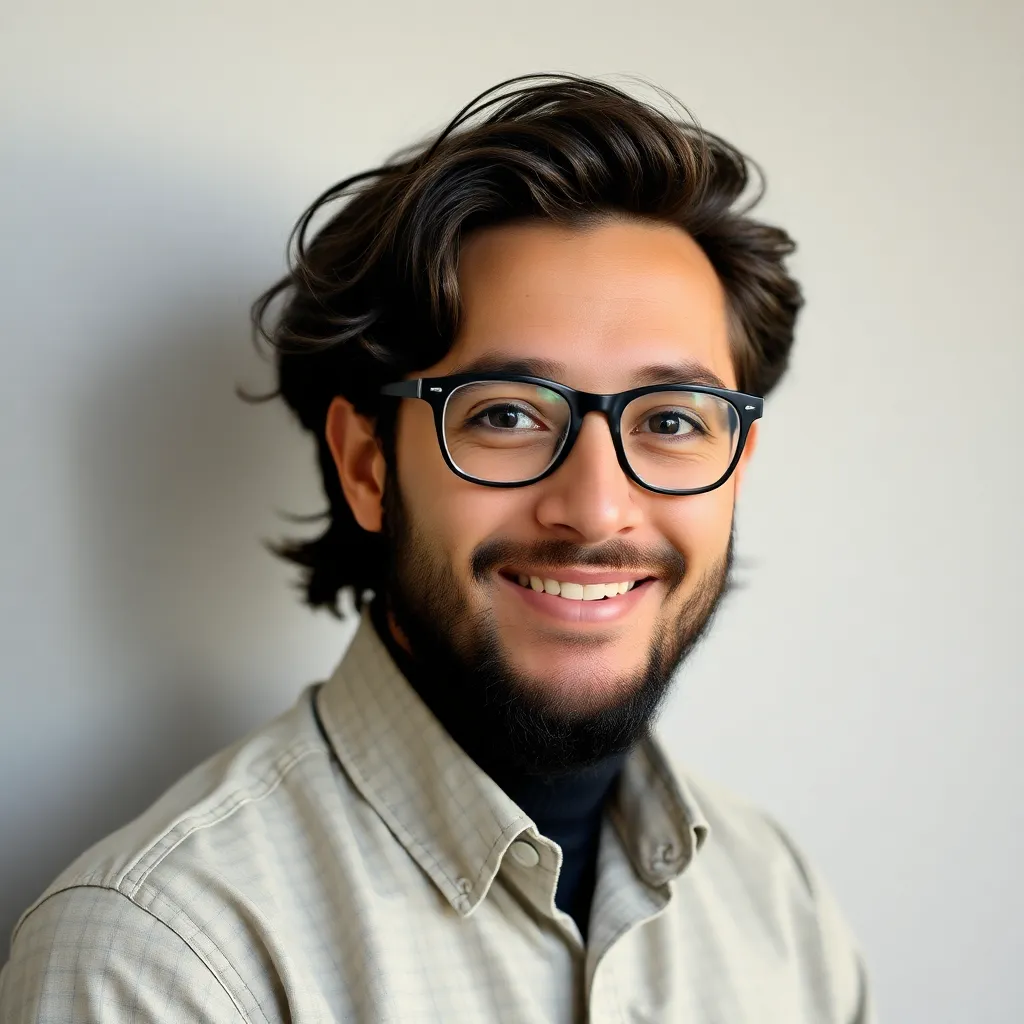
News Leon
Mar 28, 2025 · 8 min read
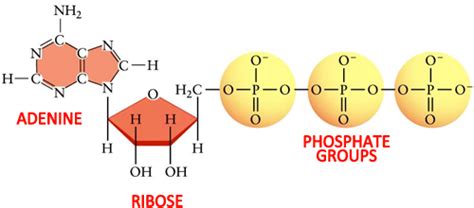
Table of Contents
Unraveling the ATP Molecule: A Deep Dive into its Three Essential Parts
Adenosine triphosphate (ATP) is the undisputed energy currency of life. This remarkable molecule fuels countless cellular processes, from muscle contraction and nerve impulse transmission to protein synthesis and DNA replication. Understanding ATP's structure is crucial to comprehending its function. This comprehensive article delves into the three fundamental parts of an ATP molecule – adenosine, ribose, and the triphosphate chain – exploring their individual properties and their synergistic contribution to ATP's vital role in biological systems.
Part 1: Adenine – The Nitrogenous Base
At the heart of the ATP molecule lies adenine, a purine base. Purines are a class of nitrogenous bases characterized by a double-ring structure, a feature crucial for their interaction with other molecules within the cell. Adenine's structure is composed of a six-membered ring fused to a five-membered ring, both containing nitrogen atoms. These nitrogen atoms are key to adenine's ability to form hydrogen bonds, vital for its interaction with other molecules, notably within the DNA double helix and in the formation of ATP itself.
Adenine's Role in ATP Function
The unique chemical properties of adenine are inextricably linked to ATP's functionality. The nitrogenous base's ability to engage in hydrogen bonding allows it to interact specifically with other molecules, such as the ribose sugar, forming a stable nucleoside. The electron distribution within the adenine ring influences its reactivity, contributing to the overall energy transfer capabilities of the ATP molecule. Without the specific structure and properties of adenine, the ATP molecule wouldn't be able to effectively store and release energy.
Adenine and Other Biological Molecules
Beyond ATP, adenine plays critical roles in other fundamental biological molecules. It's a constituent of adenosine monophosphate (AMP), adenosine diphosphate (ADP), and several crucial coenzymes, including NAD+ (nicotinamide adenine dinucleotide) and FAD (flavin adenine dinucleotide), both vital for cellular respiration. This underscores the fundamental importance of adenine in various metabolic pathways and its pivotal role in cell function. The consistency of adenine across these various molecules showcases its evolutionary significance and its fundamental importance to life.
Part 2: Ribose – The Sugar Backbone
Connected to adenine is ribose, a five-carbon sugar (pentose) with the chemical formula C₅H₁₀O₅. Unlike deoxyribose found in DNA, ribose contains a hydroxyl (-OH) group on the 2' carbon, a crucial difference influencing the structural properties and stability of RNA compared to DNA. This hydroxyl group contributes to the increased reactivity of RNA, which is fitting given RNA's involvement in numerous catalytic and regulatory roles within the cell.
Ribose's Structural Significance in ATP
In ATP, ribose acts as the backbone connecting adenine to the triphosphate group. Its specific arrangement of carbon atoms, along with the presence of hydroxyl groups, allows for the attachment of both the adenine base and the phosphate groups through stable phosphodiester bonds. The ribose sugar provides a crucial structural framework, enabling the precise spatial arrangement necessary for effective energy transfer. The positioning of the ribose sugar dictates the orientation of the phosphate groups, influencing the ease of hydrolysis and subsequent energy release.
Ribose and its Cyclical Structure
Ribose exists primarily in its cyclic form, creating a five-membered ring structure. This ring structure provides stability and contributes to the overall conformation of the ATP molecule. The precise geometry of this ring influences the angles of the bonds connecting ribose to adenine and the triphosphate chain. These angles are essential for the optimal interaction with enzymes involved in ATP synthesis and hydrolysis. Any deviation in the ribose ring structure could significantly impair ATP's function.
Part 3: Triphosphate Chain – The Energy Reservoir
The triphosphate chain is the defining characteristic of ATP and the source of its remarkable energy-storing capacity. This chain consists of three phosphate groups (PO₄³⁻) linked together by high-energy phosphoanhydride bonds. These bonds are particularly high-energy because of the proximity of the negatively charged phosphate groups and the resulting electrostatic repulsion. This repulsion is a key factor in the energy storage and release mechanism of ATP.
High-Energy Phosphoanhydride Bonds
The phosphoanhydride bonds connecting the phosphate groups in ATP are the key to the molecule's energy-storing capability. These bonds are characterized by their high free energy of hydrolysis, meaning that the energy released upon their breakage is significantly greater than that released by other types of chemical bonds. This high energy release is harnessed by the cell to drive various endergonic (energy-requiring) reactions. The hydrolysis of these bonds is a crucial aspect of ATP's functionality, powering a myriad of cellular processes.
ATP Hydrolysis and Energy Release
When ATP undergoes hydrolysis, a water molecule reacts with one of the phosphoanhydride bonds, cleaving it and releasing a phosphate group. This reaction results in the formation of adenosine diphosphate (ADP) and inorganic phosphate (Pi), releasing a significant amount of energy. This energy is then utilized by the cell to power various energy-demanding processes, such as muscle contraction, active transport, and protein synthesis. The energy released isn't directly used; instead, it's coupled to other reactions, making them thermodynamically feasible.
ATP Regeneration: The Cyclic Nature of Energy Transfer
The process of ATP hydrolysis is not a one-way street. ATP is constantly being regenerated from ADP and Pi through cellular respiration, a crucial metabolic pathway that harvests energy from the breakdown of nutrients. This continuous cycle of ATP hydrolysis and regeneration ensures a constant supply of energy for cellular functions. This cyclical nature makes ATP a remarkably efficient and sustainable energy carrier. The regeneration ensures that the cell has a consistent supply of usable energy to meet its continuous energy demands. Without this cycle, cellular function would quickly grind to a halt.
The Interplay of Adenine, Ribose, and the Triphosphate Chain
The three parts of the ATP molecule – adenine, ribose, and the triphosphate chain – work synergistically. The adenine base provides a specific recognition site for enzymes and other molecules involved in ATP metabolism. Ribose provides the structural framework for linking adenine to the triphosphate chain, ensuring the correct spatial arrangement of the phosphate groups. Finally, the triphosphate chain stores the energy, readily released upon hydrolysis. This interplay of structural components ensures ATP's efficient function as an energy carrier and is critical to life.
ATP's Universal Role in Biological Systems
ATP's importance extends beyond a single cellular process; it fuels a vast array of biological activities, showcasing its remarkable adaptability and versatility. This molecule is truly the universal energy currency, powering life across all domains of life. The efficiency of ATP in energy transfer is a testament to its exquisitely designed structure.
ATP and Cellular Respiration: The Energy-Generating Powerhouse
Cellular respiration is the central metabolic pathway responsible for generating ATP. Through a series of complex reactions involving glycolysis, the Krebs cycle, and oxidative phosphorylation, cells harvest energy from the breakdown of glucose and other nutrients. This energy is used to generate a proton gradient across the inner mitochondrial membrane, which then drives ATP synthesis by ATP synthase. The efficiency of this process is remarkable, demonstrating the crucial role of ATP in cellular energetics.
ATP and Muscle Contraction: Powering Movement
Muscle contraction, responsible for all forms of movement from locomotion to cellular transport, is highly dependent on ATP. The interaction between actin and myosin filaments, the proteins responsible for muscle contraction, is fueled by ATP hydrolysis. The energy released from ATP hydrolysis enables the conformational changes in myosin that drive the sliding filament mechanism, resulting in muscle shortening and force generation. Without the readily available energy from ATP, muscle contraction would be impossible.
ATP and Active Transport: Moving Molecules Against Concentration Gradients
Active transport, the movement of molecules against their concentration gradients, requires energy input. ATP hydrolysis provides the energy needed for various protein pumps embedded in cell membranes to transport molecules such as ions and sugars against their concentration gradients. This process is crucial for maintaining cellular homeostasis and facilitating various physiological processes. The ability to move molecules against gradients is essential for cellular function, and ATP is the primary energy source powering this process.
ATP and Protein Synthesis: Building the Cellular Machinery
Protein synthesis, the process of building proteins from amino acids, is another energy-intensive process requiring a significant amount of ATP. ATP hydrolysis drives various steps in protein synthesis, including the activation of amino acids and the translocation of ribosomes along mRNA. The faithful replication of genetic information and the subsequent production of proteins are central to life, and ATP is the vital energy source for this critical process.
ATP and Signal Transduction: Communication within the Cell
ATP also plays a significant role in signal transduction, the process of communication within and between cells. ATP can act as a signaling molecule itself, binding to specific receptors and triggering downstream signaling cascades. This role highlights the versatility of ATP, emphasizing its broader role in cellular regulation. The ability to act as both an energy source and a signaling molecule exemplifies its versatility and fundamental importance.
In conclusion, the three components of an ATP molecule – adenine, ribose, and the triphosphate chain – are intricately linked, forming a highly efficient energy storage and transfer system vital to all living organisms. Understanding the structure and function of each component offers critical insight into the myriad of biological processes powered by this remarkable molecule. The intricate interplay of these components ensures the seamless transfer and utilization of energy within the cell, fueling life itself. Further research into the details of ATP's structure and function continues to provide valuable insights into the intricacies of cellular processes and the fundamental principles of life.
Latest Posts
Latest Posts
-
What Is The Advantage Of Crossing Over
Mar 31, 2025
-
Si Unit Of Density Of Water
Mar 31, 2025
-
What Is The Molecular Geometry Of Bef2
Mar 31, 2025
-
Attached Earlobes Is A Recessive Trait In Humans
Mar 31, 2025
-
How Many Electrons Does Nitrogen Have In Its Outer Shell
Mar 31, 2025
Related Post
Thank you for visiting our website which covers about Three Parts Of An Atp Molecule . We hope the information provided has been useful to you. Feel free to contact us if you have any questions or need further assistance. See you next time and don't miss to bookmark.