Reversible And Irreversible Process In Thermodynamics
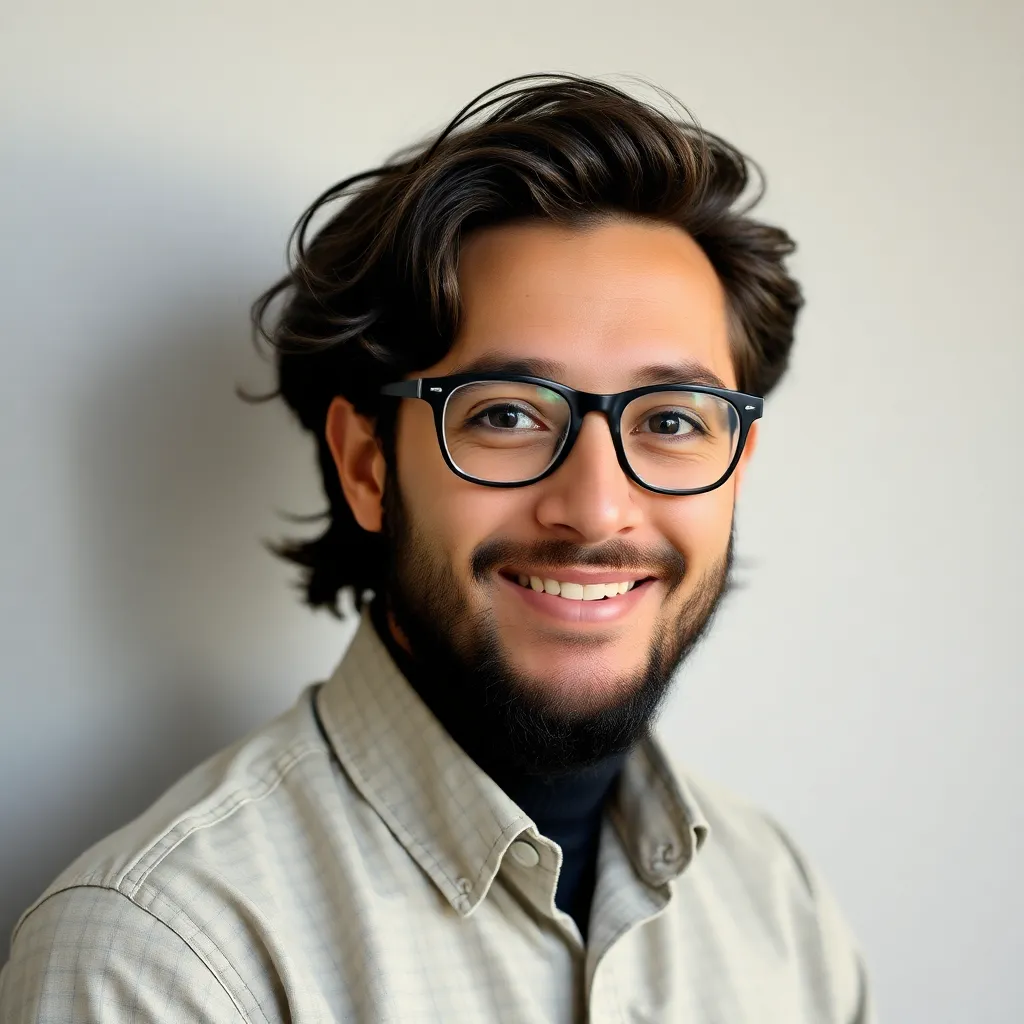
News Leon
Apr 07, 2025 · 6 min read
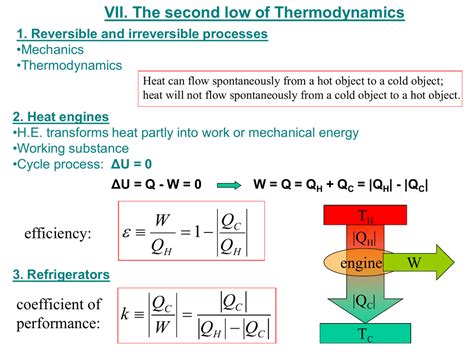
Table of Contents
Reversible and Irreversible Processes in Thermodynamics: A Comprehensive Guide
Thermodynamics, the study of energy and its transformations, rests on a fundamental distinction: the classification of processes as either reversible or irreversible. Understanding this distinction is crucial for grasping many core concepts in thermodynamics and its applications in various fields, from engineering and chemistry to biology and cosmology. This comprehensive guide delves into the intricacies of reversible and irreversible processes, exploring their defining characteristics, underlying mechanisms, and practical implications.
What is a Reversible Process?
A reversible process is an idealized theoretical process that can be reversed without leaving any trace on the surroundings. This means that both the system and its surroundings can be restored to their initial states after the process is reversed. The key here is the absence of any dissipative effects, meaning there's no loss of energy to the surroundings in the form of heat due to friction, viscosity, or other similar phenomena.
Characteristics of a Reversible Process:
- Infinitesimally Slow: A reversible process proceeds infinitely slowly, allowing the system to remain infinitesimally close to equilibrium at each stage. This ensures that there are no significant temperature or pressure gradients within the system or between the system and its surroundings.
- No Friction or Viscosity: Frictional forces and viscous effects inherently lead to energy dissipation as heat. In a reversible process, these effects are absent or negligible.
- No Heat Transfer with a Finite Temperature Difference: Heat transfer between two bodies can only occur if there's a temperature difference. A reversible process involves heat transfer that occurs across an infinitesimally small temperature difference, ensuring no energy is wasted in the transfer process.
- Equilibrium at Each Stage: At every point during a reversible process, the system is in equilibrium with its surroundings. This allows for the instantaneous reversal of the process.
Examples of (Near-)Reversible Processes:
While truly reversible processes are theoretical ideals, certain processes can closely approximate reversibility under specific conditions. These include:
- Isothermal Expansion/Compression of an Ideal Gas: If carried out slowly and carefully, with the gas in contact with a large thermal reservoir maintaining a constant temperature, expansion or compression can approach reversibility.
- Slow, Isothermal Change of Phase: The melting of ice at 0°C and 1 atm pressure, if performed slowly and without superheating or supercooling, is a near-reversible process.
- Reversible Cell: An ideal electrochemical cell operating under conditions of negligible internal resistance can closely approximate a reversible process.
What is an Irreversible Process?
An irreversible process, in contrast to a reversible process, is a real-world process that cannot be reversed without leaving some change in the system or its surroundings. These processes are characterized by an increase in entropy, signifying the dissipation of energy that cannot be recovered.
Characteristics of an Irreversible Process:
- Finite Rate of Change: Irreversible processes occur at a finite rate, causing deviations from equilibrium during the process.
- Presence of Friction and Viscosity: These dissipative forces inevitably lead to the generation of heat and a loss of usable energy.
- Heat Transfer with a Finite Temperature Difference: The transfer of heat between bodies with a finite temperature difference is inherently irreversible.
- Spontaneous Processes: Many spontaneous processes are irreversible, such as the free expansion of a gas into a vacuum, heat flow from a hot object to a cold one, and chemical reactions that proceed spontaneously.
Examples of Irreversible Processes:
Irreversible processes are the norm in the real world. Some common examples include:
- Free Expansion of a Gas: If a gas is allowed to expand rapidly into a vacuum, this is a highly irreversible process. The energy used to overcome the internal forces of the gas is not recoverable.
- Heat Transfer between Bodies at Different Temperatures: Heat spontaneously flows from a hotter body to a colder one, but the reverse process will not occur without external work.
- Mixing of Gases: Once two gases are mixed, they will not spontaneously separate into their original components.
- Chemical Reactions: Most chemical reactions are irreversible under normal conditions, progressing spontaneously towards equilibrium.
- Friction and Viscosity: Any process involving friction or viscosity, such as the sliding of two surfaces or the flow of a viscous fluid, is an irreversible process due to energy loss as heat.
- Plastic Deformation: When a solid material undergoes plastic deformation, it changes shape permanently, and this is an irreversible change.
- Combustion: Burning of fuel is a highly irreversible process where chemical energy is converted into heat and light, and the products cannot easily revert back to the reactants.
Entropy and the Second Law of Thermodynamics: The Key to Understanding Reversibility
The concept of entropy, a measure of disorder or randomness in a system, is central to understanding the distinction between reversible and irreversible processes. The Second Law of Thermodynamics states that the total entropy of an isolated system can only increase over time or remain constant in ideal cases where the system is in a steady state or undergoing a reversible process.
For a reversible process, the change in entropy of the system and its surroundings is zero (ΔS<sub>total</sub> = 0). In contrast, for an irreversible process, the total entropy always increases (ΔS<sub>total</sub> > 0). This increase in entropy reflects the dispersal of energy and the increase in disorder associated with irreversible processes. The increase in entropy is a measure of the "arrow of time" - irreversible processes always move towards states of greater disorder.
Practical Implications and Applications
The distinction between reversible and irreversible processes has profound practical implications in various fields:
- Engineering: Understanding reversibility helps engineers design more efficient systems by minimizing energy losses due to friction, heat transfer, and other irreversible processes. The Carnot cycle, a theoretical cycle representing the most efficient heat engine possible, relies on reversible processes as a benchmark for comparison.
- Chemistry: The concept of reversibility is crucial in understanding chemical reactions and equilibrium. Reversible reactions can proceed in both directions under appropriate conditions, while irreversible reactions typically proceed to completion.
- Biology: Biological systems are inherently irreversible, with metabolic processes constantly increasing entropy. Living organisms maintain a low level of entropy internally by constantly exchanging energy and matter with their surroundings.
- Cosmology: The Second Law of Thermodynamics and the inherent irreversibility of processes play a significant role in understanding the evolution of the universe.
Improving Efficiency through Minimizing Irreversibility
While completely eliminating irreversibility is impossible in practical systems, engineers and scientists constantly strive to minimize its effects to enhance efficiency. Techniques employed include:
- Reducing Friction: Utilizing lubricants, improving surface finishes, and employing hydrodynamic bearings can significantly minimize frictional losses.
- Improving Heat Transfer: Employing efficient heat exchangers and optimizing insulation can reduce energy losses through heat transfer.
- Optimizing Process Parameters: Careful control of pressure, temperature, and flow rates can minimize irreversibilities in various processes.
- Using Advanced Materials: Advanced materials with lower viscosity or enhanced heat transfer properties can help reduce energy losses.
Conclusion: The Irreversible Reality and the Reversible Ideal
Reversible processes, though theoretical ideals, serve as a crucial benchmark against which to compare and analyze real-world processes. By understanding the characteristics and implications of both reversible and irreversible processes, we gain a deeper understanding of energy transformations and the limits imposed by the Second Law of Thermodynamics. While complete reversibility remains an unattainable goal in practice, the pursuit of minimizing irreversibility remains a crucial objective in diverse fields, driving innovation and improving efficiency in numerous applications. The constant increase of entropy in the universe, reflecting the pervasive nature of irreversibility, highlights the profound impact of this fundamental concept in thermodynamics.
Latest Posts
Latest Posts
-
Which Of The Following Is True About Ecosystems
Apr 07, 2025
-
The Strongest Part Of A Magnet Is
Apr 07, 2025
-
Derivative Of Log Base 3 Of X
Apr 07, 2025
-
What Is The Lowest Energy Level Having F Orbitals
Apr 07, 2025
-
Elements In A Periodic Group Have Similar
Apr 07, 2025
Related Post
Thank you for visiting our website which covers about Reversible And Irreversible Process In Thermodynamics . We hope the information provided has been useful to you. Feel free to contact us if you have any questions or need further assistance. See you next time and don't miss to bookmark.