In Part A Of The Figure An Electron Is Shot
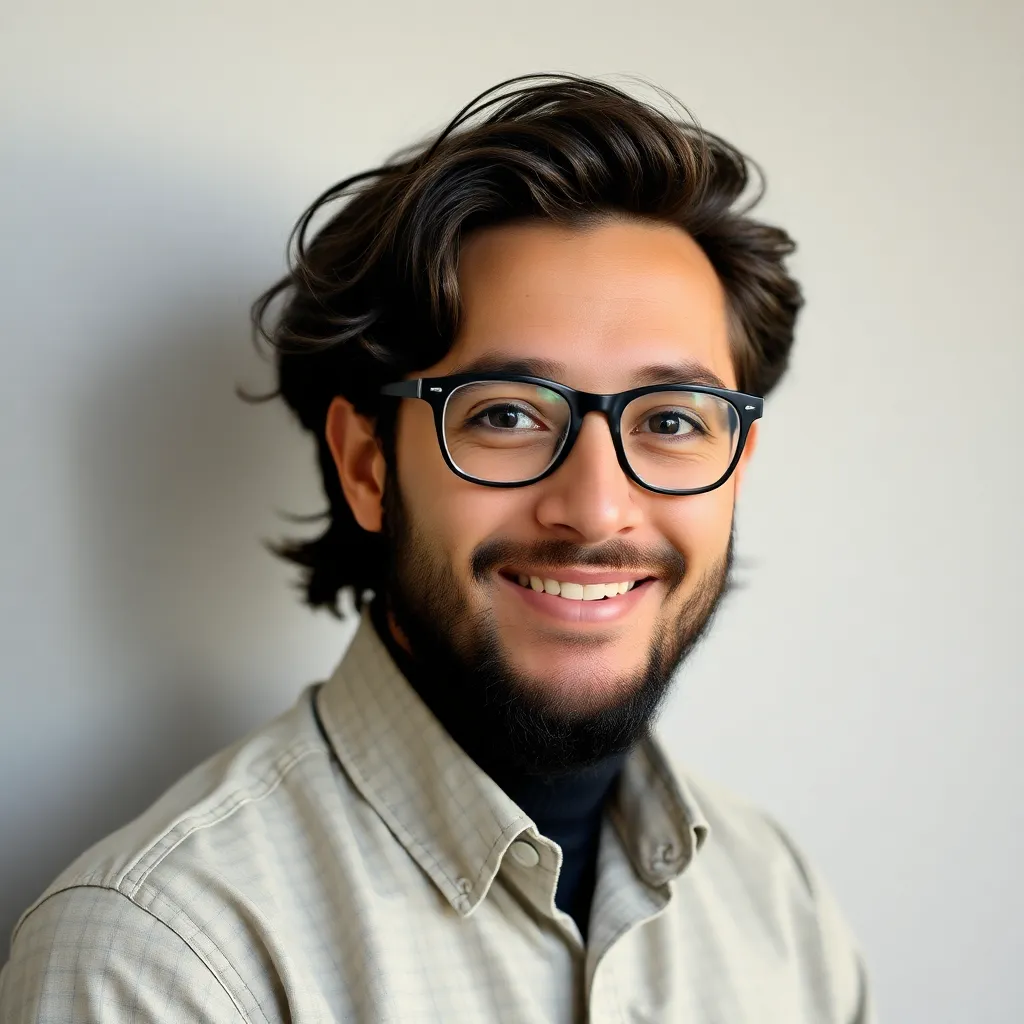
News Leon
Mar 27, 2025 · 7 min read
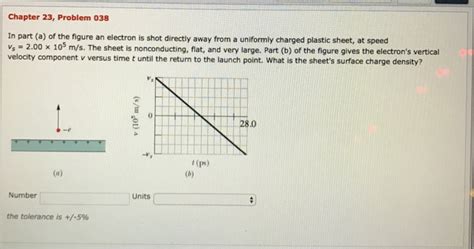
Table of Contents
Decoding the Electron's Journey: A Deep Dive into Figure Part A
The seemingly simple image of an electron being "shot" – often depicted in physics diagrams – hides a wealth of complex phenomena. Understanding the behavior of this fundamental particle under various conditions is crucial to grasping numerous scientific principles, from basic electromagnetism to the intricacies of quantum mechanics. This article will dissect the complexities of such a scenario, analyzing the forces at play, the potential outcomes, and the broader implications of this seemingly straightforward experiment.
Part A: The Setup and Initial Conditions
Before delving into the specifics of the electron's trajectory, we need to establish the context. Figure Part A, presumably depicting an experimental setup, requires careful consideration of several key factors:
1. The Electron Source:
The origin of the electron is critical. Is it emitted from a heated filament (thermionic emission), extracted from a metal surface via the photoelectric effect, or perhaps produced by a particle accelerator? The method of electron generation significantly influences its initial energy and momentum. A heated filament produces electrons with a range of energies, while photoemission yields electrons with energies dictated by the incident light frequency. Accelerators, on the other hand, can provide electrons with precisely controlled, high energies.
2. Initial Velocity and Direction:
The electron's initial velocity vector – magnitude and direction – is another crucial parameter. This directly determines its subsequent path. The direction is often depicted as a straight line, but minor deviations can significantly affect the outcome, particularly in the presence of external fields. The magnitude, directly related to the kinetic energy (1/2 * mv²), dictates the electron's interaction strength with external forces. A higher initial velocity means greater inertia, leading to less deflection by weaker fields.
3. The Surrounding Environment:
The environment surrounding the electron's path plays a crucial role. Is it a vacuum, a gas-filled chamber, or a region with a significant electromagnetic field? A vacuum minimizes interactions with other particles, simplifying analysis. A gas-filled chamber introduces the possibility of collisions with gas molecules, leading to scattering and energy loss. The presence of electromagnetic fields – electric or magnetic – profoundly influences the electron's trajectory, introducing curvature and altering its energy.
Part B: Forces Acting on the Electron
Once the initial conditions are established, we can analyze the forces acting on the electron, dictating its path. Several forces might be involved:
1. Electromagnetic Forces:
Electromagnetic forces are the dominant forces acting on charged particles like electrons. This force is described by the Lorentz force equation: F = q(E + v x B), where:
- F is the force on the electron
- q is the electron's charge (negative)
- E is the electric field vector
- v is the electron's velocity vector
- B is the magnetic field vector
- x represents the cross product
An electric field accelerates the electron along its field lines. A magnetic field, however, exerts a force perpendicular to both the electron's velocity and the magnetic field direction, causing the electron to move in a curved path. The interplay between electric and magnetic fields creates diverse and interesting trajectories.
2. Gravitational Force:
While negligible compared to electromagnetic forces, gravity still technically acts on the electron. However, its effect is generally insignificant at the scales relevant to most experiments involving individual electrons. The gravitational force is many orders of magnitude smaller than the electromagnetic forces involved, making it safely ignorable in almost all practical scenarios.
3. Collisional Forces:
If the experiment isn't performed in a vacuum, collisions with gas molecules become a significant factor. These collisions are random and inelastic, causing the electron to lose energy and change direction. The frequency and severity of these collisions depend on the gas pressure and the electron's energy. The analysis of this scenario becomes significantly more complex, often requiring statistical methods.
Part C: Possible Trajectories and Outcomes
The combination of initial conditions and acting forces determines the electron's trajectory. Let's explore a few possible scenarios:
1. Free Flight in a Vacuum:
In the absence of external fields and collisions, the electron would travel in a straight line at a constant velocity, determined by its initial conditions. This is the simplest scenario, providing a baseline for understanding more complex situations.
2. Motion in a Uniform Electric Field:
A uniform electric field will cause a constant acceleration of the electron in the direction of the field (opposite to the field direction due to the electron's negative charge). The resulting trajectory is a parabola, analogous to projectile motion under gravity. The curvature of the parabola depends on the electric field strength and the electron's initial velocity.
3. Motion in a Uniform Magnetic Field:
A uniform magnetic field exerts a force perpendicular to the electron's velocity, resulting in circular motion. The radius of this circular path is determined by the electron's momentum, charge, and the magnetic field strength. If the electron's velocity has a component parallel to the magnetic field, the resulting motion is a helix, a combination of circular and linear motion.
4. Motion in Combined Electric and Magnetic Fields (Crossed Fields):
The combination of electric and magnetic fields leads to more complex trajectories. Depending on the relative strengths and orientations of the fields, the electron might move in a straight line (if the forces cancel each other), a cycloid, or another more intricate curve. This scenario is crucial in many experimental setups, like velocity selectors in mass spectrometers.
5. Influence of Collisions:
The presence of gas molecules introduces randomness into the electron's path. The electron will undergo numerous collisions, resulting in a zig-zag trajectory. The overall direction might still be influenced by external fields, but the path becomes highly unpredictable at the microscopic level. Analyzing this situation often involves statistical methods and probability distributions.
Part D: Applications and Significance
Understanding the behavior of an electron in various scenarios is pivotal across multiple scientific disciplines:
1. Particle Accelerators:
Particle accelerators rely on precise manipulation of electric and magnetic fields to accelerate electrons (and other particles) to incredibly high energies. The design of these machines depends heavily on a detailed understanding of electron dynamics under the influence of electromagnetic fields.
2. Electron Microscopes:
Electron microscopes use beams of electrons to create high-resolution images. The focusing and manipulation of these beams depend on carefully controlled electromagnetic fields, illustrating the practical application of electron trajectory control.
3. Plasma Physics:
In plasma physics, the collective behavior of many electrons and ions is studied. Understanding individual electron motion is crucial for modeling and predicting plasma dynamics, with applications ranging from fusion energy research to space physics.
4. Semiconductor Devices:
The operation of many semiconductor devices, like transistors and integrated circuits, relies on the controlled movement of electrons within solid-state materials. Understanding electron transport in these materials requires a solid grasp of electron behavior under the influence of electric fields and lattice interactions.
5. Fundamental Physics:
Studying the motion of electrons provides insights into fundamental physics, testing theories like quantum mechanics and electromagnetism. Experiments involving precise control of electron trajectories are crucial for verifying theoretical predictions and probing the fundamental laws of nature.
Conclusion: Beyond Part A
The seemingly simple image in Figure Part A represents a gateway to a rich and complex world of physics. Analyzing the electron's trajectory requires careful consideration of numerous factors: initial conditions, interacting forces, and the surrounding environment. By understanding these elements, we can unlock a deeper comprehension of fundamental principles, enabling advancements in numerous scientific and technological fields. From the design of particle accelerators to the functioning of semiconductor devices, the controlled manipulation of electron motion plays a crucial role in our modern technological landscape. The journey of the electron, starting with the simple depiction in "Part A," leads to a vast and fascinating exploration of the physical world.
Latest Posts
Latest Posts
-
Which Of The Following Is A Variable Cost
Mar 30, 2025
-
What Type Of Bacteria Convert Ammonia To Nitrites And Nitrates
Mar 30, 2025
-
Which Of The Following Is Chemical Change
Mar 30, 2025
-
Explanation Of Dover Beach By Matthew Arnold
Mar 30, 2025
-
What Type Of Lever Is A Wheelbarrow
Mar 30, 2025
Related Post
Thank you for visiting our website which covers about In Part A Of The Figure An Electron Is Shot . We hope the information provided has been useful to you. Feel free to contact us if you have any questions or need further assistance. See you next time and don't miss to bookmark.