Dna Serves As A Template For The Synthesis Of
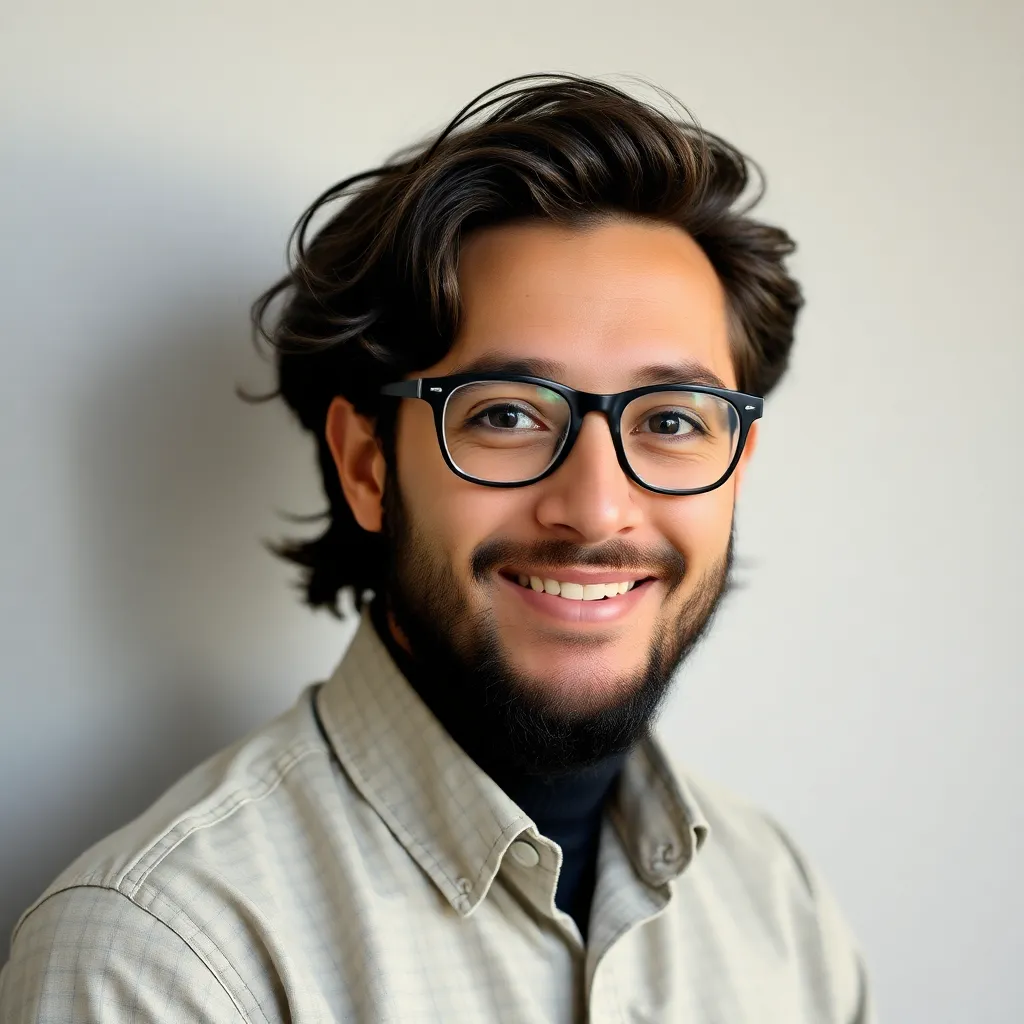
News Leon
Apr 03, 2025 · 7 min read
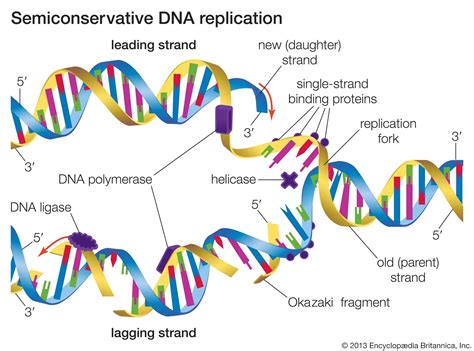
Table of Contents
DNA Serves as a Template for the Synthesis of: A Deep Dive into Replication, Transcription, and Translation
DNA, the blueprint of life, doesn't simply exist passively within a cell. Its remarkable function lies in its ability to serve as a template for the synthesis of vital molecules essential for cellular function and heredity. This process isn't a singular event but rather a sophisticated series of steps involving replication, transcription, and translation. Understanding these processes is crucial to grasping the fundamental mechanisms of life. This comprehensive article will delve into each of these processes, explaining their intricate mechanisms and highlighting their significance in the context of cellular biology and genetics.
DNA Replication: Doubling the Genetic Material
DNA replication is the process by which a cell creates an exact copy of its DNA before cell division. This ensures that each daughter cell receives a complete and identical set of genetic instructions. The process is remarkably accurate, minimizing errors that could lead to mutations and potentially harmful consequences.
The Semiconservative Model: A Key Principle
The fundamental principle of DNA replication is the semiconservative model. This model proposes that each new DNA molecule consists of one strand from the original DNA molecule (the template strand) and one newly synthesized strand. This ensures the faithful transmission of genetic information across generations.
Key Enzymes and Proteins Involved: A Molecular Orchestra
Several key enzymes and proteins orchestrate the precise and efficient replication of DNA. These include:
-
DNA Helicase: This enzyme unwinds the double helix, separating the two strands to create a replication fork. This separation is crucial as it exposes the template strands for replication.
-
Single-Stranded Binding Proteins (SSBs): These proteins bind to the separated strands, preventing them from re-annealing and maintaining the stability of the replication fork. They keep the strands apart, allowing the replication machinery to work efficiently.
-
DNA Primase: DNA polymerase, the enzyme responsible for adding nucleotides to the growing DNA strand, cannot initiate synthesis de novo. It requires a short RNA primer synthesized by DNA primase, providing a 3'-OH group to which DNA polymerase can add nucleotides.
-
DNA Polymerase: This is the workhorse enzyme of DNA replication. It adds nucleotides to the 3' end of the growing DNA strand, synthesizing a new strand complementary to the template strand. Different types of DNA polymerases have specific roles, including proofreading and repair. The accuracy of DNA polymerase is vital for maintaining the integrity of the genome. It ensures high fidelity replication by removing errors in the newly synthesised strand.
-
DNA Ligase: DNA replication proceeds discontinuously on the lagging strand, creating Okazaki fragments. DNA ligase joins these fragments together to create a continuous strand. It seals the gaps in the DNA backbone, creating a complete, unbroken strand.
-
Topoisomerases: These enzymes relieve the torsional strain ahead of the replication fork caused by the unwinding of the DNA helix. They prevent the DNA from becoming overwound or supercoiled, ensuring smooth progression of the replication process.
Leading and Lagging Strands: A Difference in Synthesis
DNA replication proceeds differently on the two strands:
-
Leading Strand: Synthesis on the leading strand is continuous, proceeding in the 5' to 3' direction towards the replication fork. This is because DNA polymerase can add nucleotides directly to the 3' end of the growing strand as the fork unwinds.
-
Lagging Strand: Synthesis on the lagging strand is discontinuous, proceeding in short fragments called Okazaki fragments. These fragments are synthesized in the 5' to 3' direction away from the replication fork. Each fragment requires a new RNA primer, and the fragments are later joined by DNA ligase.
Fidelity and Proofreading: Ensuring Accuracy
The accuracy of DNA replication is crucial for maintaining genome stability. DNA polymerase possesses proofreading capabilities, enabling it to detect and correct errors during synthesis. This proofreading activity significantly reduces the error rate, minimizing the risk of mutations. Furthermore, mismatch repair systems further refine the accuracy of replication by identifying and correcting any remaining mismatches.
Transcription: DNA to RNA
Transcription is the process of synthesizing RNA from a DNA template. This is a crucial step in gene expression, as RNA carries the genetic information from the DNA to the ribosomes, where protein synthesis takes place.
Key Players in Transcription: RNA Polymerase and Transcription Factors
Several key players are involved in transcription:
-
RNA Polymerase: This enzyme synthesizes RNA from a DNA template. It binds to the promoter region of a gene, unwinds the DNA double helix, and adds ribonucleotides to the 3' end of the growing RNA molecule.
-
Transcription Factors: These proteins regulate the binding of RNA polymerase to the promoter, influencing the rate of transcription. They are essential for controlling gene expression.
-
Promoter Region: This is a specific DNA sequence located upstream of the gene that serves as the binding site for RNA polymerase and transcription factors.
-
Terminator Sequence: This sequence signals the end of transcription. Once RNA polymerase encounters the terminator, it releases the newly synthesized RNA molecule.
Stages of Transcription: Initiation, Elongation, and Termination
Transcription proceeds in three main stages:
-
Initiation: RNA polymerase binds to the promoter region, unwinds the DNA double helix, and initiates RNA synthesis.
-
Elongation: RNA polymerase moves along the DNA template, adding ribonucleotides to the 3' end of the growing RNA molecule.
-
Termination: RNA polymerase encounters the terminator sequence and releases the newly synthesized RNA molecule. The DNA double helix rewinds.
Types of RNA: mRNA, tRNA, and rRNA
Different types of RNA molecules are synthesized during transcription, each playing a distinct role in protein synthesis:
-
Messenger RNA (mRNA): Carries the genetic information from DNA to the ribosomes.
-
Transfer RNA (tRNA): Carries amino acids to the ribosomes during translation.
-
Ribosomal RNA (rRNA): Forms a structural and catalytic component of ribosomes.
Translation: RNA to Protein
Translation is the final step in gene expression, where the genetic information encoded in mRNA is used to synthesize proteins. This process takes place in the ribosomes.
The Ribosome: The Protein Synthesis Machine
Ribosomes are complex molecular machines composed of rRNA and proteins. They provide a platform for the assembly of amino acids into polypeptide chains. They consist of two subunits, a large and a small subunit, that come together during translation.
Key Players in Translation: mRNA, tRNA, and Aminoacyl-tRNA Synthetases
Several key players are involved in translation:
-
mRNA: Carries the genetic code from DNA to the ribosomes.
-
tRNA: Carries amino acids to the ribosomes. Each tRNA molecule has a specific anticodon that recognizes a particular codon on the mRNA.
-
Aminoacyl-tRNA Synthetases: These enzymes attach the correct amino acid to its corresponding tRNA molecule. This is a crucial step to ensure the accuracy of protein synthesis. The enzyme recognises both the tRNA and the amino acid and forms a covalent bond between them.
The Genetic Code: Deciphering the Message
The genetic code is a set of rules that specifies the correspondence between codons (three-nucleotide sequences on mRNA) and amino acids. This code is nearly universal, meaning it is conserved across all living organisms. Each codon specifies a particular amino acid, with some codons serving as start or stop signals.
Stages of Translation: Initiation, Elongation, and Termination
Translation proceeds in three main stages:
-
Initiation: The ribosome binds to the mRNA molecule, and the initiator tRNA (carrying methionine) binds to the start codon (AUG).
-
Elongation: The ribosome moves along the mRNA molecule, reading each codon and adding the corresponding amino acid to the growing polypeptide chain. This involves the precise pairing of codons on the mRNA with anticodons on tRNA molecules.
-
Termination: The ribosome encounters a stop codon (UAA, UAG, or UGA), and the polypeptide chain is released. Release factors bind to the stop codon, causing the release of the polypeptide chain from the ribosome.
Post-Translational Modifications: Refining the Protein
After translation, proteins often undergo post-translational modifications, such as glycosylation, phosphorylation, or cleavage. These modifications are essential for the proper folding, function, and stability of proteins.
Conclusion: A Symphony of Molecular Processes
The synthesis of molecules from DNA is a remarkable and tightly regulated process involving a symphony of molecular events. DNA replication ensures the accurate duplication of the genome, transcription converts genetic information into RNA, and translation translates this information into proteins – the workhorses of the cell. Understanding these processes is fundamental to comprehending the complexities of life and the mechanisms underlying heredity, development, and disease. The intricate interactions between enzymes, proteins, and nucleic acids highlight the elegance and precision of cellular machinery, underscoring the power and importance of DNA as the template for life itself. Future research continues to uncover the subtleties and nuances within these processes, providing ever-increasing insights into the fundamentals of molecular biology and genetics.
Latest Posts
Latest Posts
-
Is Work A Scalar Or A Vector Quantity
Apr 04, 2025
-
Which Provides The Best Objective Summary Of This Excerpt
Apr 04, 2025
-
Classify The Following Triangle As Acute Obtuse Or Right Apex
Apr 04, 2025
-
Predict The Organic Product Of The Following Reaction
Apr 04, 2025
-
Why Is Production Possibility Curve Concave
Apr 04, 2025
Related Post
Thank you for visiting our website which covers about Dna Serves As A Template For The Synthesis Of . We hope the information provided has been useful to you. Feel free to contact us if you have any questions or need further assistance. See you next time and don't miss to bookmark.