Why Anode Is Negative In Galvanic Cell
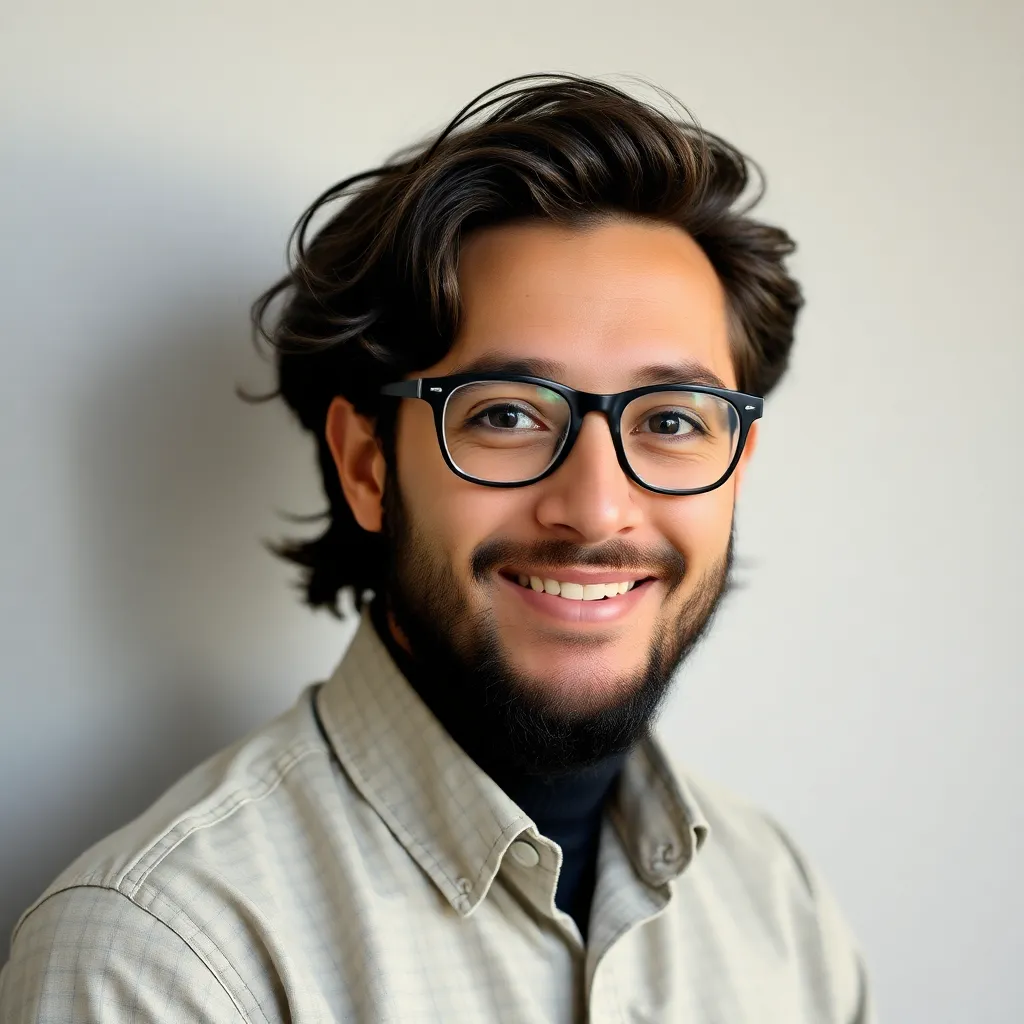
News Leon
Apr 05, 2025 · 6 min read
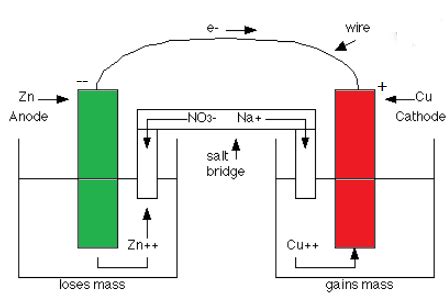
Table of Contents
Why is the Anode Negative in a Galvanic Cell? Understanding Oxidation and Electron Flow
The seemingly simple question, "Why is the anode negative in a galvanic cell?" belies a deeper understanding of fundamental electrochemical principles. This article delves into the intricacies of galvanic cells, explaining why the anode carries a negative charge and how this relates to the overall process of spontaneous redox reactions. We'll explore the concepts of oxidation, reduction, electron flow, and the conventions used in electrochemical notation, providing a comprehensive understanding accessible to both beginners and those seeking a more nuanced perspective.
Understanding Galvanic Cells: The Power of Spontaneous Reactions
A galvanic cell, also known as a voltaic cell, is an electrochemical cell that converts chemical energy into electrical energy through a spontaneous redox (reduction-oxidation) reaction. This type of cell is the foundation of many batteries we use daily. The key to its operation lies in the separation of the oxidation and reduction half-reactions, allowing the flow of electrons to generate an electrical current.
The Two Halves: Oxidation and Reduction
Every redox reaction involves two simultaneous processes:
- Oxidation: The loss of electrons by a species. The species that loses electrons is called the reducing agent because it causes the reduction of another species.
- Reduction: The gain of electrons by a species. The species that gains electrons is called the oxidizing agent because it causes the oxidation of another species.
These reactions are always coupled; one cannot occur without the other. In a galvanic cell, these half-reactions are physically separated, facilitating the harnessing of the electron flow.
The Anode: The Site of Oxidation
The anode is the electrode where oxidation occurs. This is crucial to understanding why it's negatively charged. Because oxidation involves the loss of electrons, the anode becomes negatively charged as electrons accumulate on its surface. These electrons are then driven through the external circuit to the cathode, creating the electrical current.
Visualizing the Electron Build-up
Imagine a piece of zinc metal (Zn) immersed in a solution containing zinc ions (Zn²⁺). Zinc atoms in the metal spontaneously lose two electrons to form zinc ions, which enter the solution:
Zn(s) → Zn²⁺(aq) + 2e⁻
These electrons are left behind on the zinc electrode, giving it a negative charge. This is the oxidation half-reaction taking place at the anode. The higher the concentration of Zn²⁺ ions, the more readily zinc atoms will lose electrons, increasing the negative charge on the anode.
The Cathode: The Site of Reduction
In contrast to the anode, the cathode is the electrode where reduction occurs. Reduction involves the gain of electrons. As electrons flow from the anode through the external circuit, they are consumed by the species at the cathode, resulting in a decrease in the number of electrons and a positively charged electrode.
Example: Copper(II) Ion Reduction
Let's consider a copper(II) ion solution (Cu²⁺) with a copper electrode. Copper(II) ions in solution gain two electrons from the external circuit to form solid copper, depositing on the copper electrode:
Cu²⁺(aq) + 2e⁻ → Cu(s)
This is the reduction half-reaction. The consumption of electrons at the cathode maintains a positive charge.
The Salt Bridge: Maintaining Electrical Neutrality
The crucial role of the salt bridge is often overlooked. The salt bridge, typically a U-shaped tube filled with an electrolyte solution (like potassium nitrate), connects the two half-cells. It serves two vital functions:
-
Maintaining Electrical Neutrality: The flow of electrons from the anode to the cathode would create an imbalance of charge in each half-cell if there were no salt bridge. The salt bridge allows the migration of ions to maintain electrical neutrality. Anions migrate towards the anode to balance the positive charge of the Zn²⁺ ions, while cations migrate towards the cathode to balance the negative charge from the increased concentration of anions.
-
Completing the Circuit: The salt bridge completes the electrical circuit, ensuring the continuous flow of electrons from the anode to the cathode. Without it, the build-up of charge would quickly halt the reaction.
Electron Flow and Potential Difference: The Driving Force
The spontaneous nature of a galvanic cell's reaction arises from the difference in electrode potentials. Each half-reaction has a specific potential, reflecting its tendency to undergo oxidation or reduction. The potential difference between the anode and cathode (the cell potential or electromotive force, EMF) drives the flow of electrons. The higher the potential difference, the greater the driving force for the reaction and the stronger the current produced.
Electrochemical Notation and Cell Diagrams
The convention for representing a galvanic cell uses a shorthand notation. For example, a galvanic cell with a zinc anode and a copper cathode would be represented as:
Zn(s) | Zn²⁺(aq) || Cu²⁺(aq) | Cu(s)
Where:
- The single vertical line (|) represents a phase boundary (e.g., between the solid zinc electrode and the zinc ion solution).
- The double vertical line (||) represents the salt bridge.
- The anode is always written on the left, and the cathode is on the right.
Why the Negative Sign? Conventions and Standard Reduction Potentials
The negative sign associated with the anode isn't inherently about the magnitude of the charge but rather a convention tied to standard reduction potentials. Electrochemists use a standard hydrogen electrode (SHE) as a reference point, assigning it a potential of 0 volts. Electrode potentials are then measured relative to the SHE.
A positive standard reduction potential indicates that the reduction half-reaction is favored (i.e., it has a greater tendency to gain electrons), while a negative standard reduction potential signifies that the oxidation half-reaction is favored. Since the anode is where oxidation occurs, it is assigned a negative potential relative to the cathode in a galvanic cell. This convention helps us predict the direction of electron flow and the overall cell potential.
Practical Applications and Further Considerations
The principles of galvanic cells underpin countless technologies, including:
- Batteries: From small button cells to large car batteries, all rely on spontaneous redox reactions to generate electricity.
- Fuel Cells: These cells convert the chemical energy of fuels (like hydrogen) directly into electrical energy, offering high efficiency and low emissions.
- Corrosion Protection: Understanding galvanic principles is crucial in designing corrosion protection systems, like sacrificial anodes.
Further considerations regarding galvanic cells include:
- Concentration effects: The concentration of ions in the half-cells affects the cell potential. The Nernst equation describes this relationship.
- Temperature dependence: The cell potential also depends on temperature.
- Overpotential: In real-world cells, there are often overpotential losses that reduce the actual cell potential.
Conclusion: A Comprehensive Understanding
The negativity of the anode in a galvanic cell is a direct consequence of the oxidation half-reaction occurring there, where electrons are released and accumulate on the electrode's surface. This fundamental principle, coupled with the reduction at the cathode and the role of the salt bridge, drives the spontaneous flow of electrons, generating the electrical energy that powers numerous technological applications. By understanding the underlying concepts of oxidation, reduction, electron flow, and the conventions of electrochemical notation, we gain a complete appreciation for the functioning of galvanic cells and their significance in our technological world. Further exploration into the nuances of electrode potentials, concentration effects, and other related factors provides an even deeper understanding of this essential electrochemical process.
Latest Posts
Latest Posts
-
The End Product Of The Calvin Cycle Is
Apr 06, 2025
-
10 Protons 11 Neutrons 10 Electrons
Apr 06, 2025
-
What Number Is 6 More Than 7
Apr 06, 2025
-
Sulfur Number Of Protons Neutrons And Electrons
Apr 06, 2025
-
Which Best Describes The Law Of Independent Assortment
Apr 06, 2025
Related Post
Thank you for visiting our website which covers about Why Anode Is Negative In Galvanic Cell . We hope the information provided has been useful to you. Feel free to contact us if you have any questions or need further assistance. See you next time and don't miss to bookmark.