During Glycolysis Glucose Is Broken Down Into Two Molecules Of
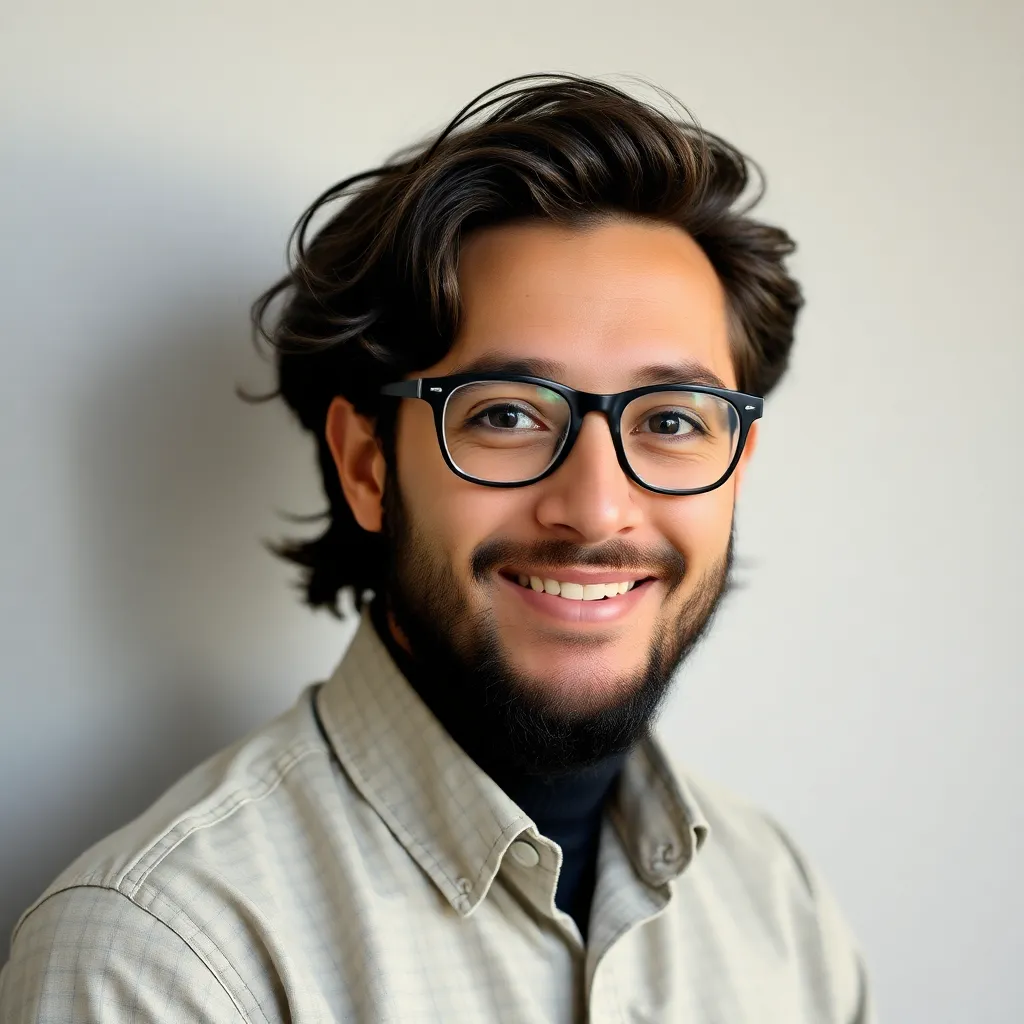
News Leon
Apr 02, 2025 · 7 min read
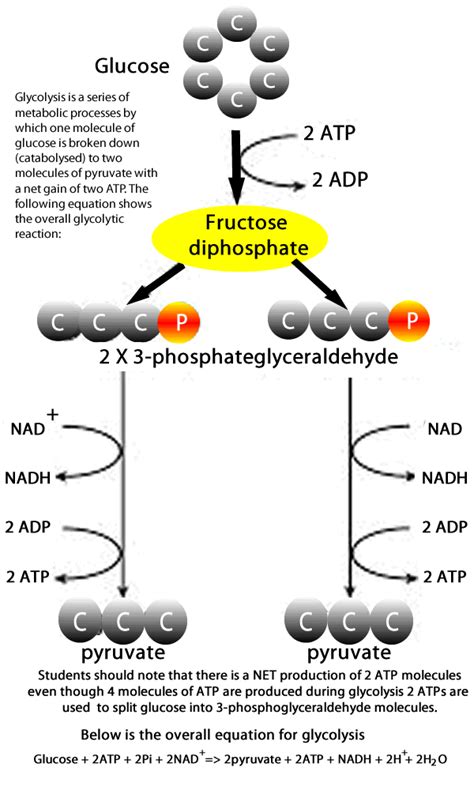
Table of Contents
During Glycolysis, Glucose is Broken Down into Two Molecules of Pyruvate: A Deep Dive into the Process
Glycolysis, the cornerstone of cellular respiration, is a fundamental metabolic pathway that breaks down glucose, a six-carbon sugar, into two molecules of pyruvate, a three-carbon compound. This process, occurring in the cytoplasm of nearly all living cells, serves as the initial stage of both aerobic (oxygen-present) and anaerobic (oxygen-absent) respiration, providing the crucial building blocks for subsequent energy production. Understanding glycolysis is vital to comprehending how cells generate energy and the intricate interplay of metabolic pathways within the body.
The Ten Steps of Glycolysis: A Detailed Breakdown
Glycolysis is a remarkably efficient process, comprising ten distinct enzymatic reactions, each carefully orchestrated to extract energy from glucose. These steps can be broadly categorized into two phases: the energy investment phase and the energy payoff phase.
Phase 1: The Energy Investment Phase (Steps 1-5)
This initial phase requires energy input to prepare glucose for subsequent breakdown. Two ATP molecules are consumed in this phase, but this investment pays off handsomely in the second phase.
1. Glucose Phosphorylation (Hexokinase): The process begins with the phosphorylation of glucose, converting it into glucose-6-phosphate. This reaction, catalyzed by hexokinase, utilizes one ATP molecule, making glucose-6-phosphate a more reactive molecule, trapping it within the cell. This crucial first step commits glucose to the glycolytic pathway.
2. Isomerization (Phosphoglucose Isomerase): Glucose-6-phosphate is then isomerized into fructose-6-phosphate by phosphoglucose isomerase. This isomerization reaction converts the aldose (glucose-6-phosphate) into a ketose (fructose-6-phosphate), a crucial structural change that prepares the molecule for the next step.
3. Second Phosphorylation (Phosphofructokinase): The second phosphorylation step is the commitment step of glycolysis, catalyzed by phosphofructokinase (PFK). This enzyme uses another ATP molecule to phosphorylate fructose-6-phosphate, forming fructose-1,6-bisphosphate. PFK is a highly regulated enzyme, acting as a control point for the entire glycolytic pathway.
4. Cleavage (Aldolase): Fructose-1,6-bisphosphate is then cleaved by aldolase into two three-carbon molecules: glyceraldehyde-3-phosphate (G3P) and dihydroxyacetone phosphate (DHAP). These two molecules are isomers of each other.
5. Isomerization (Triose Phosphate Isomerase): DHAP is rapidly and reversibly isomerized into G3P by triose phosphate isomerase. This ensures that both molecules produced in step 4 contribute to the subsequent energy-generating steps. From this point onwards, the pathway proceeds with two molecules of G3P.
Phase 2: The Energy Payoff Phase (Steps 6-10)
This phase sees the generation of ATP and NADH, the reduced form of nicotinamide adenine dinucleotide, a crucial electron carrier involved in energy production. For each molecule of glucose, this phase yields a net gain of four ATP molecules and two NADH molecules.
6. Oxidation and Phosphorylation (Glyceraldehyde-3-Phosphate Dehydrogenase): G3P undergoes oxidation and phosphorylation in a reaction catalyzed by glyceraldehyde-3-phosphate dehydrogenase. This reaction involves the transfer of electrons to NAD+, reducing it to NADH, and the addition of an inorganic phosphate group to G3P, forming 1,3-bisphosphoglycerate. This is a crucial step where energy is captured in the high-energy phosphate bond.
7. Substrate-Level Phosphorylation (Phosphoglycerate Kinase): 1,3-bisphosphoglycerate then donates its high-energy phosphate group to ADP, forming ATP through substrate-level phosphorylation. This reaction, catalyzed by phosphoglycerate kinase, generates one ATP molecule for each G3P molecule (two ATP molecules per glucose). The product is 3-phosphoglycerate.
8. Isomerization (Phosphoglycerate Mutase): 3-phosphoglycerate is isomerized into 2-phosphoglycerate by phosphoglycerate mutase. This isomerization positions the phosphate group for the next crucial step.
9. Dehydration (Enolase): 2-phosphoglycerate undergoes dehydration, catalyzed by enolase, removing a water molecule and forming phosphoenolpyruvate (PEP). This creates a high-energy phosphate bond.
10. Substrate-Level Phosphorylation (Pyruvate Kinase): Finally, PEP transfers its high-energy phosphate group to ADP, yielding another ATP molecule through substrate-level phosphorylation. This reaction, catalyzed by pyruvate kinase, produces pyruvate, the end product of glycolysis.
The Net Yield of Glycolysis: Energy Accounting
After the completion of glycolysis, the net yield per glucose molecule is:
- 2 ATP molecules: Although four ATP molecules are produced, two were consumed in the energy investment phase.
- 2 NADH molecules: These electron carriers are crucial for subsequent energy production in aerobic respiration.
- 2 Pyruvate molecules: These three-carbon molecules serve as the starting point for the next stages of cellular respiration, namely the citric acid cycle and oxidative phosphorylation.
Regulation of Glycolysis: A Fine-Tuned Process
Glycolysis is a tightly regulated process, ensuring that glucose is metabolized efficiently and in accordance with the cell's energy needs. Key regulatory enzymes, particularly hexokinase, phosphofructokinase (PFK), and pyruvate kinase, are subject to allosteric regulation, meaning their activity is modulated by binding of specific molecules.
-
Hexokinase: Is inhibited by its product, glucose-6-phosphate. High levels of glucose-6-phosphate indicate sufficient glucose metabolism, slowing down the initial step.
-
Phosphofructokinase (PFK): This is the primary regulatory enzyme of glycolysis. It is allosterically activated by ADP and AMP (indicating low energy levels) and inhibited by ATP and citrate (indicating high energy levels). This ensures that glycolysis only proceeds when energy is needed.
-
Pyruvate Kinase: Is activated by fructose-1,6-bisphosphate (a product of an earlier step) and inhibited by ATP and alanine (an amino acid).
These regulatory mechanisms ensure a balanced and efficient flow of glucose through the glycolytic pathway.
The Fate of Pyruvate: Aerobic vs. Anaerobic Respiration
The fate of pyruvate depends on the availability of oxygen.
Aerobic Respiration: The Citric Acid Cycle and Oxidative Phosphorylation
In the presence of oxygen, pyruvate enters the mitochondria, where it is converted into acetyl-CoA, entering the citric acid cycle (Krebs cycle). This cycle further oxidizes pyruvate, generating more ATP, NADH, and FADH2 (another electron carrier). These electron carriers then fuel oxidative phosphorylation, the electron transport chain, generating a large amount of ATP through chemiosmosis. This is the most efficient way to extract energy from glucose.
Anaerobic Respiration: Fermentation
In the absence of oxygen, pyruvate undergoes fermentation, a process that regenerates NAD+ from NADH, allowing glycolysis to continue. There are two main types of fermentation:
-
Lactic acid fermentation: Pyruvate is reduced to lactate, regenerating NAD+. This occurs in muscle cells during strenuous exercise and in some microorganisms.
-
Alcoholic fermentation: Pyruvate is converted into ethanol and carbon dioxide, regenerating NAD+. This process is used by yeast and some bacteria.
Fermentation produces only a small amount of ATP compared to aerobic respiration, but it allows cells to continue generating energy under anaerobic conditions.
Glycolysis and its Significance in Various Biological Processes
Glycolysis is not merely a pathway for energy production; it plays a crucial role in various other biological processes:
-
Biosynthesis: Intermediates of glycolysis serve as precursors for the biosynthesis of various essential molecules, including amino acids, fatty acids, and nucleotides.
-
Gluconeogenesis: This is the process of synthesizing glucose from non-carbohydrate sources, such as amino acids and glycerol. Several glycolytic enzymes also participate in gluconeogenesis.
-
Disease: Disruptions in glycolysis can contribute to various diseases, including cancer, diabetes, and genetic disorders affecting enzyme activity.
-
Medicine: Understanding glycolysis is crucial for developing drugs targeting metabolic pathways and treating metabolic disorders.
Conclusion: A Fundamental Process with Far-Reaching Implications
Glycolysis, the process of breaking down glucose into two molecules of pyruvate, is a fundamental metabolic pathway essential for life. Its ten enzymatic steps, carefully regulated to meet the cell's energy demands, represent a marvel of biochemical efficiency. The fate of pyruvate and the subsequent energy production pathways – aerobic respiration or fermentation – determine the ultimate energy yield. Moreover, glycolysis plays a broader role in cellular metabolism, contributing to biosynthesis and serving as a crucial component in various biological processes and disease mechanisms. A thorough understanding of glycolysis is vital in various fields, from medicine to biotechnology. Further research continues to illuminate the intricacies of this fundamental process and its implications for human health and disease.
Latest Posts
Latest Posts
-
Find The Area Of Scalene Triangle
Apr 03, 2025
-
Which Of The Following Is True Of Muscle Contraction
Apr 03, 2025
-
Elements That Are Liquids At Room Temperature
Apr 03, 2025
-
Direction Of Propagation Of Electromagnetic Waves
Apr 03, 2025
-
How Many Protons Neutrons And Electrons In Iron
Apr 03, 2025
Related Post
Thank you for visiting our website which covers about During Glycolysis Glucose Is Broken Down Into Two Molecules Of . We hope the information provided has been useful to you. Feel free to contact us if you have any questions or need further assistance. See you next time and don't miss to bookmark.