A Denatured Protein Has Lost Its
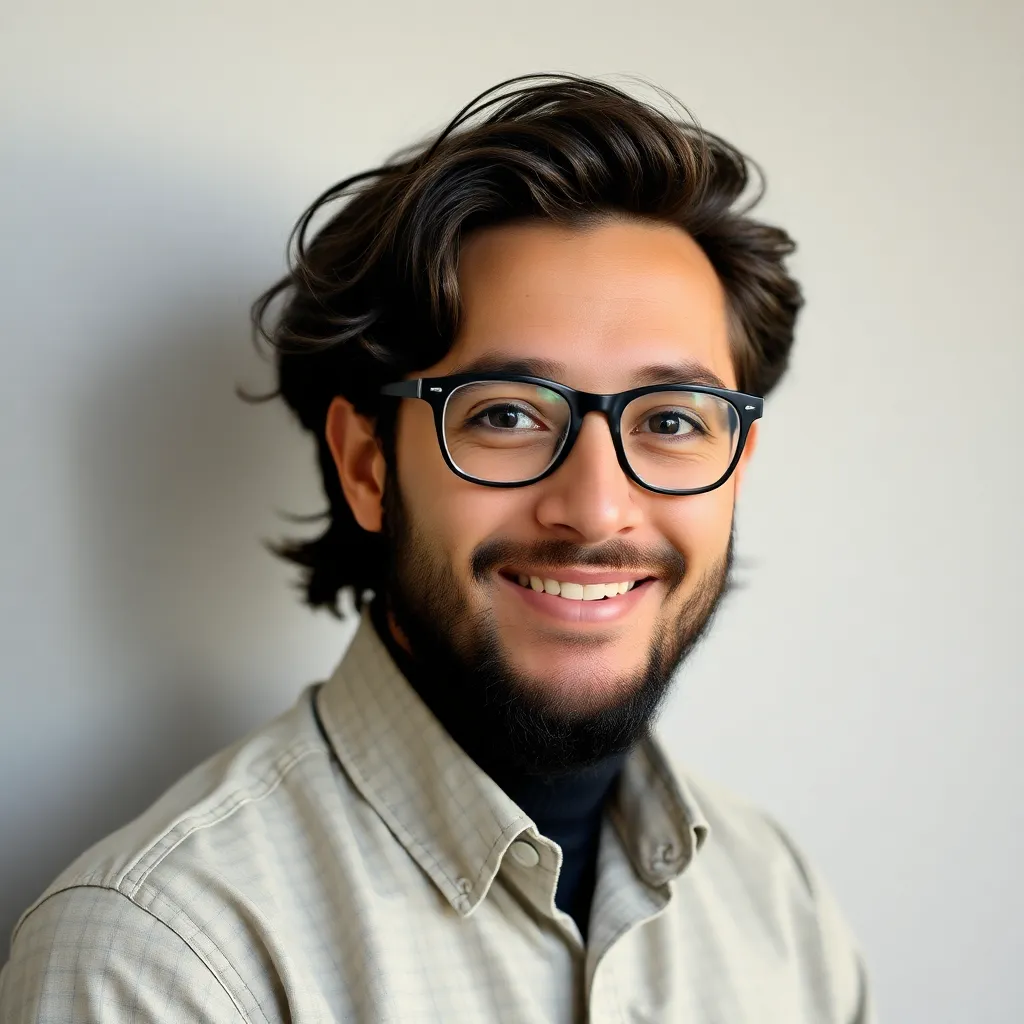
News Leon
Apr 04, 2025 · 6 min read
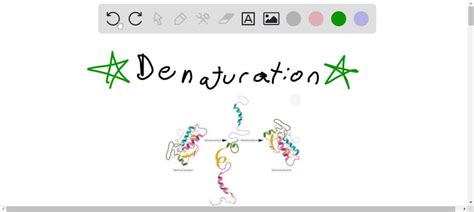
Table of Contents
A Denatured Protein Has Lost Its: Structure, Function, and the Path to Recovery
Proteins are the workhorses of the cell, carrying out a vast array of crucial functions. Their ability to perform these functions hinges critically on their three-dimensional structure, a precisely folded arrangement dictated by their amino acid sequence. When a protein loses this intricate structure, a process known as denaturation, it loses its functionality. This article delves deep into the consequences of protein denaturation, exploring the types of structural changes involved, the factors that trigger denaturation, and the potential for protein refolding and recovery.
What is Protein Denaturation?
Protein denaturation is the partial or complete unfolding of a protein's three-dimensional structure. This unfolding disrupts the protein's native conformation, which is the specific three-dimensional arrangement it adopts under physiological conditions. The native conformation is essential for a protein's function, as it positions crucial amino acid residues in specific locations to facilitate interactions with other molecules (substrates, ligands, etc.) or to create active sites for enzymatic activity. When a protein is denatured, these critical interactions and active sites are lost or significantly compromised.
Levels of Protein Structure Affected by Denaturation
Protein structure is hierarchical, encompassing four levels:
-
Primary Structure: This refers to the linear sequence of amino acids in a polypeptide chain. Denaturation generally does not affect the primary structure; the peptide bonds remain intact.
-
Secondary Structure: This involves local folding patterns like alpha-helices and beta-sheets stabilized by hydrogen bonds between backbone atoms. Denaturation often disrupts these secondary structures, causing the helices and sheets to unravel.
-
Tertiary Structure: This is the overall three-dimensional arrangement of a polypeptide chain, encompassing interactions between amino acid side chains (e.g., disulfide bridges, hydrophobic interactions, ionic bonds, hydrogen bonds). Denaturation extensively disrupts tertiary structure, causing the protein to lose its compact, folded shape.
-
Quaternary Structure: This applies to proteins composed of multiple polypeptide subunits. Denaturation can lead to the dissociation of these subunits, further compromising the protein's function.
Factors that Cause Protein Denaturation
Several factors can induce protein denaturation, each disrupting the weak interactions that maintain the protein's native conformation:
1. Heat:
High temperatures provide sufficient kinetic energy to overcome the weak interactions (hydrogen bonds, hydrophobic interactions, etc.) that stabilize the protein's structure. This leads to the unfolding and loss of function. Egg whites, for instance, turn white when heated due to the denaturation of albumen proteins.
2. pH Changes:
Extreme pH values (both highly acidic and highly alkaline) can alter the charges on amino acid side chains, disrupting electrostatic interactions that contribute to the protein's three-dimensional structure. Changes in charge can also interfere with hydrogen bonding and disrupt the hydrophobic core.
3. Chemical Denaturants:
Certain chemicals, such as urea and guanidine hydrochloride, act as denaturants by disrupting hydrogen bonds and hydrophobic interactions. Urea, for example, can interfere with hydrogen bonding by competing for hydrogen bond donors and acceptors.
4. Chaotropic Agents:
These agents, including sodium dodecyl sulfate (SDS) and trifluoroethanol (TFE), disrupt the hydrophobic interactions that stabilize the protein core. SDS, a detergent, binds to hydrophobic regions of the protein, effectively unfolding it.
5. Mechanical Stress:
Agitation, shearing forces, or high pressure can physically unfold proteins by disrupting their delicate structure. This can be particularly important in industrial processes that involve protein handling and processing.
6. Organic Solvents:
Organic solvents, such as ethanol and acetone, can disrupt the hydrophobic interactions within the protein, leading to denaturation. These solvents compete with the protein for interactions with water molecules, thus destabilizing the hydrophobic core.
Consequences of Protein Denaturation
The loss of a protein's native conformation directly impacts its function. Denatured proteins often exhibit:
-
Loss of biological activity: Enzymes lose their catalytic activity, transport proteins lose their ability to bind and carry molecules, and structural proteins lose their mechanical strength.
-
Increased susceptibility to proteolytic degradation: Denatured proteins become more vulnerable to proteases, enzymes that break down proteins. This is because the exposed peptide bonds are now more accessible to the proteases' active sites.
-
Aggregation and precipitation: Denatured proteins may aggregate, forming insoluble clumps or precipitates. This can be problematic in various settings, including in biological systems (leading to disease) and industrial processes (causing clogging or fouling).
-
Changes in immunological properties: The alteration in protein structure can affect its interaction with antibodies, potentially leading to changes in antigenicity.
Protein Refolding and Renaturation
Under certain conditions, some denatured proteins can regain their native structure and function, a process known as renaturation or refolding. This highlights the crucial role of the amino acid sequence in dictating the protein's three-dimensional structure. The information necessary for correct folding is inherently encoded within the primary sequence.
Several factors influence the success of protein refolding:
-
The nature of the denaturant: Removing the denaturant gradually can increase the chances of successful refolding.
-
The protein's inherent stability: Some proteins are more prone to refolding than others due to their intrinsic stability and the strength of their various internal interactions.
-
The presence of chaperone proteins: Chaperone proteins assist in the correct folding of newly synthesized or denatured proteins, preventing aggregation and misfolding. They often work by binding to partially unfolded proteins, shielding them from aggregation, and guiding them towards the correct native conformation.
-
Environmental conditions: The optimal conditions for refolding are often similar to the physiological conditions in which the protein normally functions (temperature, pH, ionic strength).
Practical Implications of Protein Denaturation
Understanding protein denaturation is crucial in numerous fields:
-
Food science: Heat treatment of food denatures proteins, affecting texture, taste, and nutritional value. The denaturation of egg proteins during cooking is a classic example.
-
Biotechnology: Protein purification and engineering often involve manipulation of denaturation and refolding conditions to isolate, modify, and study proteins.
-
Medicine: Protein denaturation plays a role in various diseases, including Alzheimer's and Parkinson's disease where protein misfolding and aggregation contribute to the pathology.
-
Cosmetology: The use of heat styling on hair leads to the denaturation of keratin proteins, resulting in changes in hair texture.
The Importance of Protein Structure Maintenance: A Cellular Perspective
The cellular environment is highly regulated to maintain optimal conditions for protein function. Maintaining proper protein folding is essential for cellular homeostasis, with failures leading to various cellular dysfunctions. The cell employs several mechanisms to prevent or mitigate protein denaturation, including:
-
Molecular chaperones: These proteins assist in protein folding and prevent aggregation. Hsp70 and Hsp60 are examples of prominent chaperones.
-
Protein disulfide isomerases: These enzymes catalyze the formation and breakage of disulfide bonds, ensuring the correct disulfide bonding pattern in proteins.
-
Proteasomal degradation: Misfolded or irreversibly denatured proteins are targeted for degradation by the proteasome, a cellular machinery responsible for protein quality control.
Disruptions to any of these mechanisms can lead to protein misfolding, aggregation, and cellular stress.
Conclusion: A Multifaceted Process with Significant Implications
Protein denaturation, though seemingly a simple process, is a complex phenomenon with significant implications across various biological and technological fields. Understanding the mechanisms of protein denaturation, the factors that trigger it, and the potential for refolding is essential for advancing our knowledge in numerous areas, from medicine and biotechnology to food science and cosmetology. Future research into protein folding and stability will undoubtedly continue to unveil new insights into this fundamental biological process, ultimately leading to improved therapeutic strategies, advanced biotechnological applications, and a deeper understanding of cellular processes. The ongoing exploration of protein denaturation underscores the importance of this process in maintaining cellular function and overall health.
Latest Posts
Latest Posts
-
Lewis Dot Diagram For Fluoride Ion
Apr 05, 2025
-
13 Protons 14 Neutrons And 10 Electrons
Apr 05, 2025
-
After Mitosis How Many Chromosomes Are In Each Daughter Cell
Apr 05, 2025
-
What Are The Products Of The Following Reaction
Apr 05, 2025
-
The Patella Is An Example Of Which Type Of Bone
Apr 05, 2025
Related Post
Thank you for visiting our website which covers about A Denatured Protein Has Lost Its . We hope the information provided has been useful to you. Feel free to contact us if you have any questions or need further assistance. See you next time and don't miss to bookmark.