Why Does Carbon Form Covalent Bonds
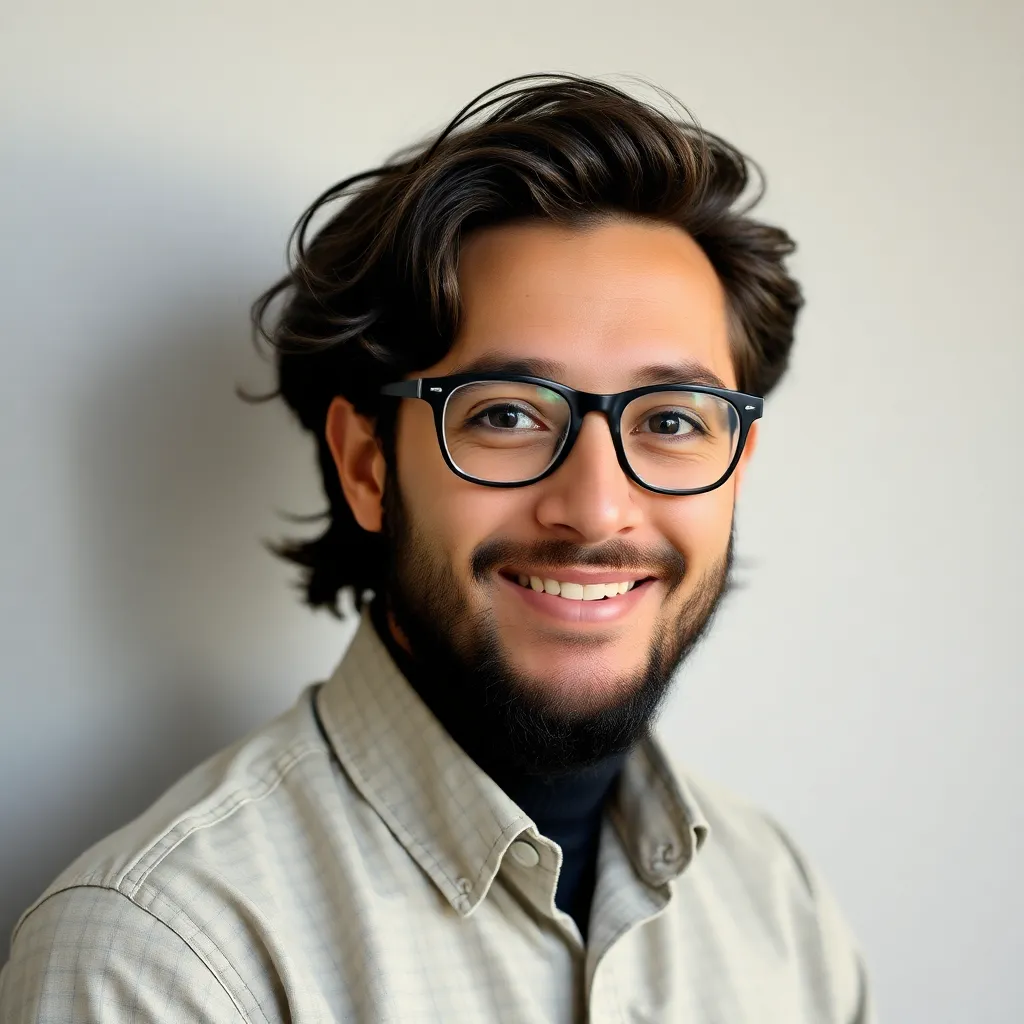
News Leon
Apr 27, 2025 · 6 min read

Table of Contents
Why Does Carbon Form Covalent Bonds? Unveiling the Secrets of Carbon's Bonding Behavior
Carbon, the cornerstone of life and a fundamental element in countless materials, exhibits a unique bonding preference: it almost exclusively forms covalent bonds. Understanding why carbon favors covalent bonding is crucial to grasping its remarkable versatility and the diversity of organic and inorganic compounds it creates. This in-depth exploration will delve into the electronic structure of carbon, the nature of covalent bonds, and the factors that contribute to carbon's overwhelming preference for this type of bonding.
Carbon's Electronic Structure: The Foundation of Covalent Bonding
At the heart of carbon's covalent bonding behavior lies its electronic configuration. Carbon possesses six electrons, arranged as follows: two in the inner shell (1s²), and four in the outer valence shell (2s²2p²). This valence shell configuration is key. It's neither particularly close to a full octet (eight electrons, providing exceptional stability) nor easily inclined to lose or gain four electrons to achieve a stable noble gas configuration. This "in-between" state makes it energetically unfavorable for carbon to form ionic bonds.
Ionic Bonds: A Less Favorable Option for Carbon
Ionic bonds involve the complete transfer of electrons from one atom to another, creating ions with opposite charges that are then attracted to each other through electrostatic forces. For carbon to achieve a stable octet through ionic bonding, it would need to either gain four electrons (becoming a highly negatively charged ion, C⁴⁻) or lose four electrons (becoming a highly positively charged ion, C⁴⁺). Both scenarios are energetically extremely unfavorable.
-
High ionization energy: Losing four electrons requires a significant amount of energy to overcome the attraction between the positively charged nucleus and the electrons. Carbon's relatively high ionization energy makes this process energetically expensive.
-
High electron affinity (but still unfavorable): While carbon does have a moderate electron affinity (the energy change associated with gaining an electron), gaining four electrons to form C⁴⁻ would result in a highly unstable, negatively charged ion due to strong electron-electron repulsions. The energy released by gaining these four electrons wouldn't compensate for the energy required to overcome these repulsive forces.
Covalent Bonds: The Energetically Favorable Choice
Covalent bonds, on the other hand, involve the sharing of electrons between atoms. This allows carbon to achieve a stable octet without the need for a large energy input or the creation of highly unstable ions. By sharing electrons with other atoms, carbon effectively completes its outer electron shell, fulfilling the octet rule and gaining stability.
This shared electron pair is attracted to the positively charged nuclei of both participating atoms, creating a strong bond that holds the atoms together. This process is energetically far more favorable than the extreme ionic possibilities.
The Versatility of Carbon's Covalent Bonding: Single, Double, and Triple Bonds
Carbon's capacity to form single, double, and triple covalent bonds further contributes to its exceptional versatility. These different bond types arise from the various ways carbon can share its four valence electrons.
Single Covalent Bonds: The Foundation of Chain Formation
A single covalent bond is formed by sharing one pair of electrons between two atoms. In the case of carbon, this allows it to form long chains and branched structures, which are fundamental to organic chemistry. The relatively free rotation around single bonds allows for flexibility in the shapes of molecules, leading to a vast array of possible structures.
Double and Triple Covalent Bonds: Enhancing Stability and Reactivity
Double covalent bonds involve the sharing of two pairs of electrons between two atoms. These bonds are stronger and shorter than single bonds, resulting in a more rigid molecular structure. Similarly, triple covalent bonds, involving the sharing of three pairs of electrons, are even stronger and shorter than double bonds.
The presence of double and triple bonds often introduces significant reactivity into molecules, as these bonds are more susceptible to breaking and forming new bonds in chemical reactions. This is crucial for the diversity of chemical transformations that are possible in organic chemistry.
Carbon's Ability to Catonate: Forming Rings and Complex Structures
Another key factor contributing to carbon's extensive bonding capacity is its ability to catenate. Catenation refers to the self-linking of atoms of the same element to form chains and rings. Carbon's ability to catenate is far superior to most other elements, largely due to the strength of the carbon-carbon bond.
This remarkable property allows carbon to form an immense variety of structures, from simple linear chains to complex branched structures and intricate ring systems. The possibility of these diverse structures further contributes to the vast number of carbon-containing compounds.
The Role of Hybridization in Carbon's Bonding
To fully understand carbon's covalent bonding, we must also consider the concept of orbital hybridization. Hybridization is the mixing of atomic orbitals within an atom to form new hybrid orbitals that are more suited for bonding. Carbon's ability to undergo hybridization plays a significant role in its bonding behavior.
sp³ Hybridization: Tetrahedral Geometry
In many organic compounds, carbon undergoes sp³ hybridization. This involves mixing one s orbital and three p orbitals to create four sp³ hybrid orbitals. These orbitals are arranged in a tetrahedral geometry, which is crucial for the formation of stable tetrahedral structures such as methane (CH₄) and diamond.
sp² Hybridization: Trigonal Planar Geometry
In compounds containing double bonds, carbon often undergoes sp² hybridization. This involves mixing one s orbital and two p orbitals to create three sp² hybrid orbitals and leaving one p orbital unhybridized. The three sp² hybrid orbitals are arranged in a trigonal planar geometry, with the unhybridized p orbital perpendicular to the plane. This configuration is essential for the formation of planar molecules like ethene (C₂H₄).
sp Hybridization: Linear Geometry
In compounds containing triple bonds, carbon undergoes sp hybridization. This involves mixing one s orbital and one p orbital to create two sp hybrid orbitals, leaving two p orbitals unhybridized. The two sp hybrid orbitals are arranged linearly, with the two unhybridized p orbitals perpendicular to the line. This configuration is necessary for the formation of linear molecules like ethyne (C₂H₂).
The Influence of Electronegativity and Bond Polarity
While carbon primarily forms covalent bonds, the electronegativity of the atoms it bonds with influences the polarity of the resulting bond. Electronegativity is the ability of an atom to attract electrons in a chemical bond.
Carbon has an intermediate electronegativity. When it bonds with atoms of similar electronegativity (such as hydrogen or carbon itself), the resulting bonds are essentially nonpolar covalent bonds. However, when carbon bonds with atoms of significantly different electronegativity (such as oxygen or nitrogen), the resulting bonds become polar covalent bonds. The unequal sharing of electrons creates a partial positive charge (δ+) on carbon and a partial negative charge (δ-) on the more electronegative atom. This polarity influences the reactivity and properties of the molecule.
Conclusion: The Remarkable Versatility of Carbon's Covalent Bonding
The overwhelming preference of carbon for covalent bonding stems from its electronic configuration, which makes ionic bonding highly unfavorable. The ability to form single, double, and triple bonds, combined with its unique ability to catenate and undergo hybridization, allows carbon to construct a vast array of intricate and diverse molecules, forming the backbone of organic chemistry and contributing to the astonishing complexity of life itself. Understanding this fundamental bonding behavior is crucial for comprehending the properties and reactivity of a countless number of compounds, from simple hydrocarbons to complex biomolecules. The versatility of carbon's covalent bonding is, undeniably, one of the most remarkable aspects of its chemistry, underpinning the rich tapestry of organic and inorganic materials that shape our world.
Latest Posts
Latest Posts
-
What Are The End Products Of Starch Digestion
Apr 27, 2025
-
The Oily Waxy Secretion Called Sebum Is Produced By
Apr 27, 2025
-
Why Human Unable To Digest Cellulose
Apr 27, 2025
-
Is Nucleotide A Monomer Or Polymer
Apr 27, 2025
-
Finding The Variance Of A Probability Distribution
Apr 27, 2025
Related Post
Thank you for visiting our website which covers about Why Does Carbon Form Covalent Bonds . We hope the information provided has been useful to you. Feel free to contact us if you have any questions or need further assistance. See you next time and don't miss to bookmark.