Which Of The Following Represents Beta Decay
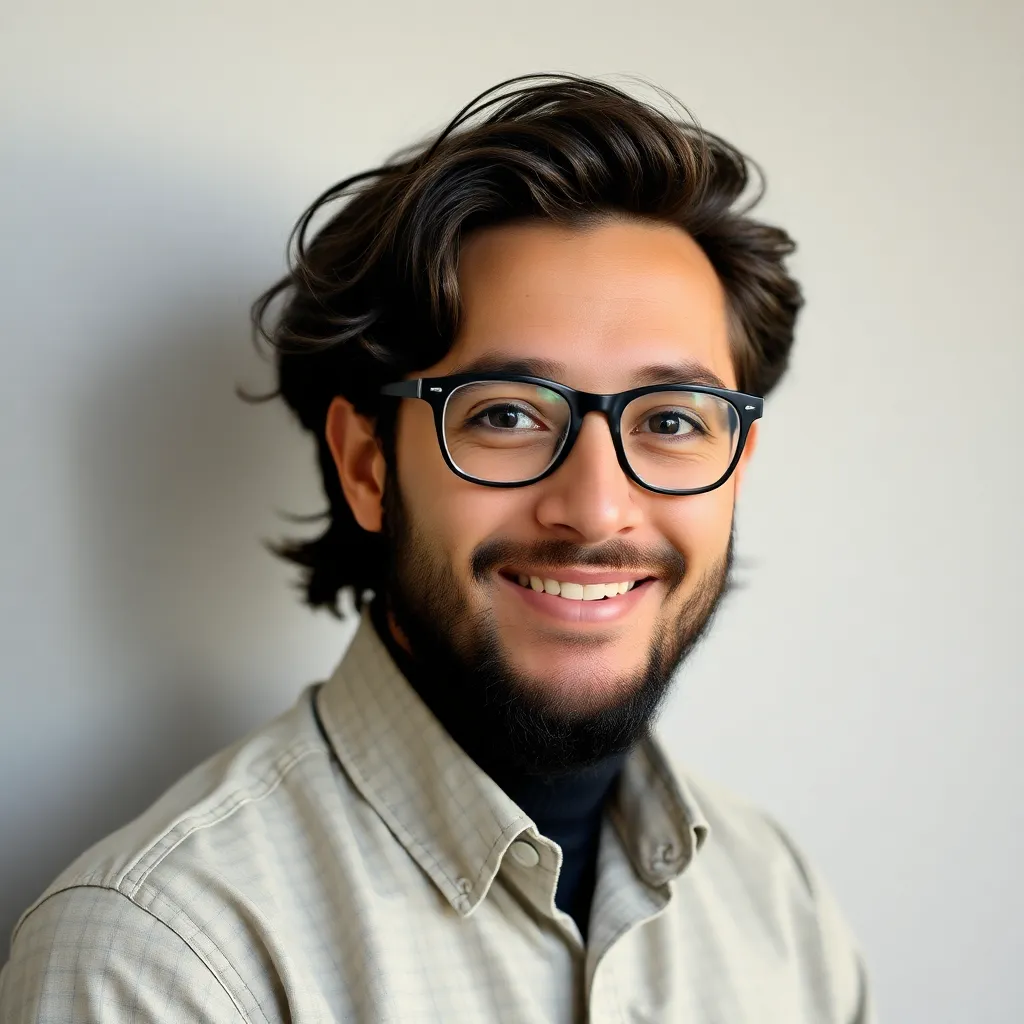
News Leon
Mar 30, 2025 · 5 min read
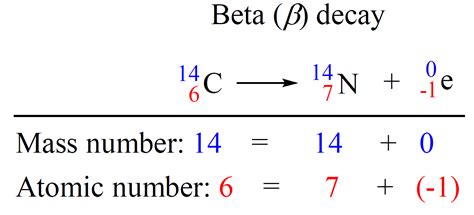
Table of Contents
Which of the following represents beta decay? Understanding Beta Decay and its Variations
Beta decay is a type of radioactive decay in which a beta particle (a high-energy electron or positron) is emitted from an atomic nucleus. This process fundamentally alters the nucleus's composition, changing its atomic number and impacting its stability. Understanding the nuances of beta decay is crucial for comprehending nuclear physics and its applications in various fields. This article delves deep into beta decay, exploring its different types, the underlying mechanisms, and how to identify it from other radioactive decay processes.
Understanding the Basics of Beta Decay
Beta decay is a fundamental process in nuclear physics where a nucleus transitions to a lower energy state by emitting a beta particle. This particle is either an electron (β⁻ decay) or a positron (β⁺ decay). This emission is accompanied by the release of a neutrino or antineutrino, ensuring the conservation of lepton number.
Key Characteristics of Beta Decay:
- Changes in Atomic Number: Beta decay alters the atomic number (Z) of the nucleus. β⁻ decay increases Z by one, while β⁺ decay decreases Z by one.
- Mass Number Remains Constant: The mass number (A), representing the total number of protons and neutrons, remains unchanged during beta decay.
- Energy Release: Beta decay releases energy in the form of kinetic energy carried by the beta particle and the neutrino.
- Weak Nuclear Force: Beta decay is governed by the weak nuclear force, one of the four fundamental forces in nature.
Beta Minus Decay (β⁻ Decay)
In β⁻ decay, a neutron within the nucleus transforms into a proton, emitting an electron (β⁻) and an electron antineutrino (ν̅ₑ). This can be represented by the following equation:
n → p + β⁻ + ν̅ₑ
where:
- n represents a neutron
- p represents a proton
- β⁻ represents an electron (beta particle)
- ν̅ₑ represents an electron antineutrino
This type of decay increases the atomic number by one while leaving the mass number unchanged. A classic example is the decay of carbon-14 into nitrogen-14:
¹⁴C → ¹⁴N + β⁻ + ν̅ₑ
Beta Plus Decay (β⁺ Decay)
In β⁺ decay, a proton within the nucleus transforms into a neutron, emitting a positron (β⁺) and an electron neutrino (νₑ). The equation is:
p → n + β⁺ + νₑ
where:
- p represents a proton
- n represents a neutron
- β⁺ represents a positron (beta particle)
- νₑ represents an electron neutrino
This type of decay decreases the atomic number by one, again keeping the mass number constant. A common example is the decay of carbon-11 into boron-11:
¹¹C → ¹¹B + β⁺ + νₑ
Electron Capture
Electron capture is a less common type of beta decay where an inner-shell electron is captured by a proton in the nucleus, transforming the proton into a neutron and emitting an electron neutrino:
p + e⁻ → n + νₑ
This process doesn't directly involve the emission of a beta particle but leads to a similar outcome: a decrease in the atomic number by one, with the mass number remaining unchanged.
Identifying Beta Decay: Key Indicators
Distinguishing beta decay from other types of radioactive decay, like alpha decay or gamma decay, requires careful observation and analysis of the emitted particles and changes in the nucleus.
Key indicators of beta decay include:
- Emission of a beta particle (electron or positron): The detection of either an electron or a positron is a definitive sign of beta decay.
- Change in atomic number but not mass number: The atomic number increases by one in β⁻ decay and decreases by one in β⁺ decay or electron capture, while the mass number remains constant.
- Presence of a neutrino or antineutrino: These elusive particles are also emitted during beta decay, though their detection is more challenging.
- Continuous energy spectrum of beta particles: Unlike alpha particles that have a discrete energy spectrum, beta particles exhibit a continuous energy spectrum because the energy is shared between the beta particle and the neutrino.
Differentiating Beta Decay from Other Decay Types:
- Alpha Decay: Alpha decay involves the emission of an alpha particle (two protons and two neutrons), resulting in a decrease of both atomic number (by two) and mass number (by four).
- Gamma Decay: Gamma decay involves the emission of a gamma ray (high-energy photon), which doesn't change the atomic number or mass number; it simply represents a transition of the nucleus to a lower energy state.
Examples of Beta Decay and their Significance
Numerous isotopes undergo beta decay, influencing various aspects of the natural world and technological applications. Let's examine a few crucial examples:
1. Carbon-14 Dating: The beta decay of carbon-14 (¹⁴C) is fundamental to radiocarbon dating, a technique used to determine the age of organic materials. The decay of ¹⁴C follows a known half-life, allowing scientists to estimate the time elapsed since the organism died.
2. Potassium-40 Decay: Potassium-40 (⁴⁰K), a naturally occurring isotope in the human body, undergoes beta decay, emitting both beta particles and gamma rays. This decay contributes to the natural background radiation we experience.
3. Iodine-131 in Medical Applications: Iodine-131 (¹³¹I) is a radioactive isotope used in nuclear medicine for the diagnosis and treatment of thyroid disorders. Its beta decay provides a targeted radiation source for destroying cancerous thyroid cells.
4. Betavoltaic Devices: Beta decay can be harnessed to generate electricity in betavoltaic devices. These devices utilize the energy released during beta decay to produce a small electric current, making them potentially useful in long-life, low-power applications.
Conclusion: The Importance of Understanding Beta Decay
Beta decay is a complex yet fundamental process in nuclear physics. Understanding its various forms, mechanisms, and implications is crucial for advancements in several scientific and technological areas, including:
- Nuclear medicine: Radioactive isotopes undergoing beta decay are used extensively in medical diagnosis and treatment.
- Archaeology and Geology: Radiocarbon dating, based on beta decay of carbon-14, is a cornerstone of dating ancient artifacts and geological formations.
- Nuclear power: Beta decay is involved in the radioactive decay chains within nuclear reactors and in the production of nuclear waste.
- Fundamental Physics: Studying beta decay contributes to our understanding of the weak nuclear force and the fundamental building blocks of matter.
This article has provided a comprehensive overview of beta decay, equipping readers with the knowledge to identify it, understand its variations, and appreciate its significance across numerous scientific disciplines. By grasping the core concepts discussed here, one can better understand the complexities of nuclear physics and its diverse applications in our world. Further research into specific isotopes and their decay processes can enhance this knowledge and provide a deeper appreciation for the fundamental processes shaping our universe.
Latest Posts
Latest Posts
-
Which Of The Following Forms A Molecular Solid
Apr 01, 2025
-
What Is The Least Electronegative Element
Apr 01, 2025
-
What Is Not A Product Of Photosynthesis
Apr 01, 2025
-
D Dx X 1 X 2
Apr 01, 2025
-
Energy For Muscle Contraction Is Most Directly Supplied By
Apr 01, 2025
Related Post
Thank you for visiting our website which covers about Which Of The Following Represents Beta Decay . We hope the information provided has been useful to you. Feel free to contact us if you have any questions or need further assistance. See you next time and don't miss to bookmark.