When An Electron Moves From A To B
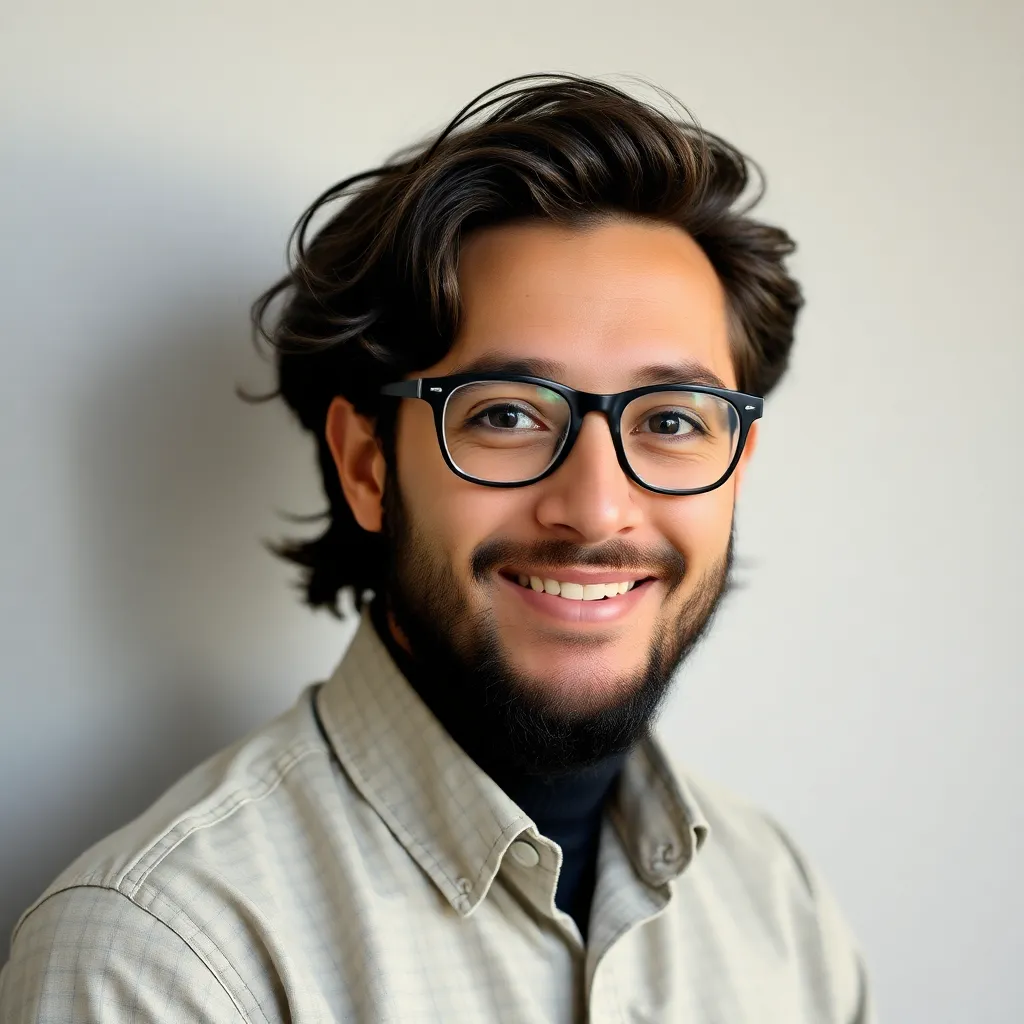
News Leon
Mar 13, 2025 · 6 min read

Table of Contents
When an Electron Moves from A to B: A Deep Dive into Quantum Mechanics
The seemingly simple question, "When an electron moves from point A to point B," unravels into a complex tapestry woven from the threads of quantum mechanics. It's a question that challenges our classical intuition and reveals the bizarre, yet beautiful, nature of the subatomic world. This exploration will delve into the intricacies of electron behavior, examining its wave-particle duality, the probabilistic nature of its movement, and the implications for our understanding of the universe.
Beyond Classical Physics: The Wave-Particle Duality of Electrons
In the classical world, we envision objects moving along well-defined trajectories. A ball thrown from point A will follow a predictable arc to point B. Electrons, however, refuse to adhere to such simplistic descriptions. This is because electrons exhibit wave-particle duality, a fundamental concept in quantum mechanics.
The Wave Nature of Electrons
Electrons aren't just tiny particles; they also possess wave-like properties. This was famously demonstrated by the double-slit experiment, where electrons, fired one at a time at a screen with two slits, created an interference pattern—a hallmark of wave behavior. This pattern wouldn't be observed if electrons were simply particles following straight paths. Instead, each electron seems to pass through both slits simultaneously, interfering with itself.
This wave-like behavior is described by the wave function, denoted by Ψ (psi). The wave function provides a probabilistic description of the electron's location. The square of the wave function, |Ψ|², gives the probability density of finding the electron at a particular point in space. It's crucial to understand that we cannot know the electron's exact path; we only know the probability of finding it at various locations.
The Particle Nature of Electrons
Despite their wave-like nature, electrons also exhibit particle-like properties. They possess specific quantities of charge and mass, and they can interact with other particles like individual entities. This dual nature is a central paradox of quantum mechanics, highlighting the limitations of our classical understanding.
The act of observing an electron's location, for instance, forces it to "choose" a specific point in space, collapsing its wave function. This wave function collapse is a controversial aspect of quantum mechanics, sparking ongoing debates about the role of measurement and observation in the quantum world.
The Uncertainty Principle: Defining the Limits of Knowledge
Heisenberg's uncertainty principle further complicates our understanding of electron movement. It states that there's a fundamental limit to the precision with which certain pairs of physical properties of a particle, like position and momentum, can be known simultaneously. The more accurately we know an electron's position, the less accurately we know its momentum (and vice-versa).
This uncertainty isn't a result of limitations in our measurement technology; it's an inherent property of the quantum world. It implies that the concept of a precise trajectory for an electron is inherently meaningless. We can only speak of probabilities.
Quantum Tunneling: The Unexpected Leap
Electrons can even overcome energy barriers that would be insurmountable according to classical physics. This phenomenon is known as quantum tunneling. Imagine an electron encountering a potential energy barrier – a region of higher energy that it should be unable to cross based on its energy level. According to quantum mechanics, there's a non-zero probability that the electron will "tunnel" through the barrier, appearing on the other side.
Quantum tunneling is crucial for many physical processes, including nuclear fusion in stars and the operation of many modern electronic devices. It highlights the fundamentally probabilistic nature of quantum mechanics and the limitations of classical intuition.
Describing Electron Movement: Schrödinger's Equation
The behavior of electrons is mathematically described by the Schrödinger equation, a fundamental equation in quantum mechanics. This equation governs the evolution of the wave function over time. Solving the Schrödinger equation for a given system allows us to calculate the probability of finding an electron at different locations and energy levels.
The solutions to the Schrödinger equation are often expressed as wave functions, which provide a probabilistic description of the electron's state. These wave functions are characterized by quantum numbers, which specify the electron's energy, angular momentum, and other properties.
The Role of Potential Energy: Shaping the Electron's Journey
The potential energy landscape significantly influences an electron's movement. An electron will tend to occupy regions of lower potential energy. For example, in an atom, electrons are attracted to the positively charged nucleus, and they occupy orbitals that minimize their potential energy. The shape and structure of these orbitals determine the probabilities of finding the electron at different locations within the atom.
Electron Movement in Different Contexts
The specifics of electron movement depend heavily on the context. Let's consider a few scenarios:
Electron Movement in Atoms
Within an atom, electrons occupy specific energy levels or orbitals. Transitions between these levels involve the absorption or emission of photons (light particles). The electron doesn't move in a continuous trajectory but rather jumps between discrete energy states.
Electron Movement in Conductors
In conductors, electrons are relatively free to move throughout the material. Their movement is influenced by an applied electric field, which causes a net flow of electrons, creating an electric current. However, even in conductors, the movement of individual electrons is still probabilistic and subject to the uncertainty principle.
Electron Movement in Semiconductors
Semiconductors exhibit intermediate electrical conductivity between conductors and insulators. The movement of electrons in semiconductors is crucial for the operation of transistors and other semiconductor devices. Doping (adding impurities) can significantly influence the electron's behavior in semiconductors.
Implications and Applications
Our understanding of electron movement has far-reaching implications across various scientific and technological fields. It's crucial for:
- Electronics: The design and function of transistors, integrated circuits, and other electronic devices depend fundamentally on our understanding of electron behavior.
- Chemistry: Chemical bonding and reactions are governed by the interactions between electrons in different atoms and molecules.
- Materials Science: The properties of materials are largely determined by the behavior of their constituent electrons.
- Nuclear Physics: Nuclear reactions and processes, such as radioactive decay, involve the movement and interaction of electrons and other subatomic particles.
Conclusion: The Ongoing Mystery of Electron Motion
The question of "When an electron moves from A to B" remains a profound and complex issue. While we have developed sophisticated mathematical tools and models to describe electron behavior, the fundamentally probabilistic nature of quantum mechanics challenges our classical intuition. The wave-particle duality, the uncertainty principle, and quantum tunneling highlight the bizarre yet beautiful characteristics of the subatomic world.
Continued research and exploration are essential for furthering our understanding of electron behavior and harnessing its power for technological advancements. The journey to fully grasp the intricacies of electron movement is an ongoing adventure, pushing the boundaries of human knowledge and understanding of the universe.
Latest Posts
Related Post
Thank you for visiting our website which covers about When An Electron Moves From A To B . We hope the information provided has been useful to you. Feel free to contact us if you have any questions or need further assistance. See you next time and don't miss to bookmark.