When A Nerve Fiber Is Polarized The Concentration Of
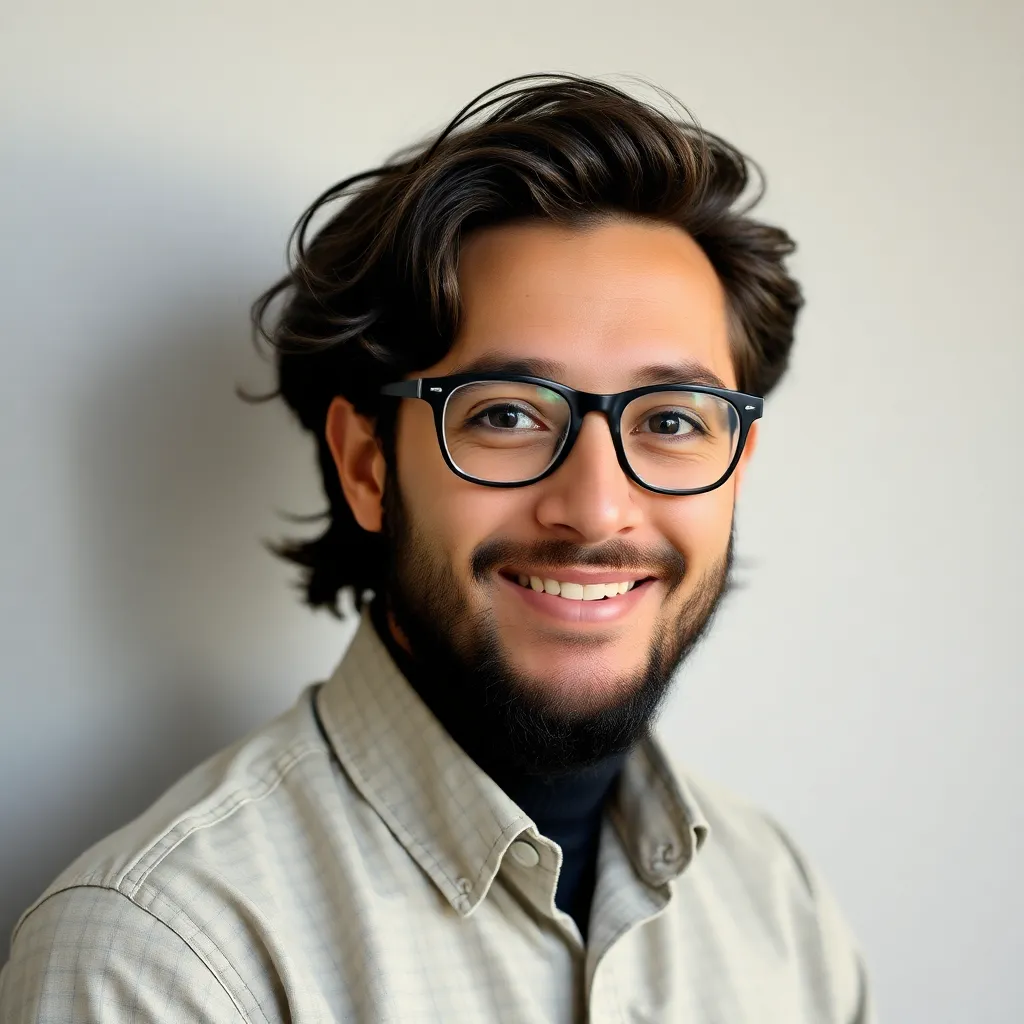
News Leon
Apr 04, 2025 · 7 min read

Table of Contents
When a Nerve Fiber is Polarized: The Concentration of Ions and the Generation of the Resting Membrane Potential
The human nervous system, a marvel of biological engineering, relies on the precise movement of ions across neuronal membranes to transmit information. At the heart of this process lies the resting membrane potential (RMP), a critical state where the neuron maintains a negative electrical charge inside the cell relative to the outside. Understanding this polarization, specifically the ionic concentration gradients responsible, is fundamental to grasping how nerve impulses are generated and transmitted.
The Crucial Role of Ion Channels
The neuronal membrane isn't just a passive barrier; it's a highly selective gatekeeper. This selectivity stems from the presence of ion channels, specialized protein complexes embedded within the lipid bilayer. These channels are exquisitely sensitive, allowing only certain ions to pass through while barring others. The types and numbers of open ion channels at any given moment determine the membrane's permeability to different ions, directly influencing the RMP.
Specifically, the RMP is significantly influenced by the permeability and concentration gradients of sodium (Na⁺), potassium (K⁺), and chloride (Cl⁻) ions. While other ions play minor roles, these three are the major players in establishing and maintaining the resting state.
The Concentration Gradients: An Unequal Distribution
The key to understanding the RMP lies in recognizing the unequal distribution of ions across the neuronal membrane. At rest, the concentration of potassium ions (K⁺) is significantly higher inside the neuron than outside. Conversely, the concentration of sodium ions (Na⁺) is much higher outside the neuron than inside. This difference isn't arbitrary; it's actively maintained by energy-consuming mechanisms, primarily the sodium-potassium pump (Na⁺/K⁺-ATPase).
This pump, a transmembrane protein, uses the energy derived from ATP hydrolysis to transport three sodium ions (Na⁺) out of the cell for every two potassium ions (K⁺) it pumps in. This constant pumping maintains the concentration gradients, even against the natural tendency of ions to diffuse down their concentration gradients.
The distribution of chloride ions (Cl⁻) is also important. While the concentration of Cl⁻ is generally higher outside the neuron, its contribution to the RMP is less dominant than that of Na⁺ and K⁺ due to the relatively low permeability of the membrane to chloride at rest.
The Role of Membrane Permeability: Selectively Letting Ions Through
Membrane permeability is crucial; it's not just about the ion concentrations, but also how easily those ions can cross the membrane. At rest, the neuronal membrane is significantly more permeable to potassium ions (K⁺) than to sodium ions (Na⁺). This higher permeability to K⁺ is largely due to the presence of numerous leak potassium channels, which remain open even at rest. These channels allow potassium ions to passively diffuse down their concentration gradient, flowing out of the cell.
The outward movement of positively charged potassium ions leaves behind a net negative charge inside the cell, contributing to the negative RMP. While some sodium ions do leak into the cell through a few open sodium channels, their contribution is less significant due to the membrane's lower permeability to sodium at rest.
Calculating the Resting Membrane Potential: The Goldman-Hodgkin-Katz Equation
The precise value of the RMP, typically around -70 millivolts (mV), isn't solely determined by the potassium equilibrium potential. The Goldman-Hodgkin-Katz (GHK) equation provides a more accurate calculation, considering the relative permeabilities of potassium, sodium, and chloride ions:
Vₘ = RT/F * ln[(P<sub>K</sub>[K⁺]<sub>out</sub> + P<sub>Na</sub>[Na⁺]<sub>out</sub> + P<sub>Cl</sub>[Cl⁻]<sub>in</sub>)/(P<sub>K</sub>[K⁺]<sub>in</sub> + P<sub>Na</sub>[Na⁺]<sub>in</sub> + P<sub>Cl</sub>[Cl⁻]<sub>out</sub>)]
Where:
- Vₘ is the membrane potential
- R is the ideal gas constant
- T is the temperature in Kelvin
- F is the Faraday constant
- P<sub>K</sub>, P<sub>Na</sub>, P<sub>Cl</sub> are the permeabilities of potassium, sodium, and chloride ions, respectively
- [K⁺]<sub>out</sub>, [Na⁺]<sub>out</sub>, [Cl⁻]<sub>out</sub> are the extracellular concentrations of potassium, sodium, and chloride ions
- [K⁺]<sub>in</sub>, [Na⁺]<sub>in</sub>, [Cl⁻]<sub>in</sub> are the intracellular concentrations of potassium, sodium, and chloride ions
This equation demonstrates that the RMP is a complex interplay between the concentration gradients of multiple ions and the membrane's permeability to each. The higher permeability to potassium, despite the relatively smaller concentration gradient compared to sodium, is the primary reason the RMP is negative.
Maintaining the Resting Membrane Potential: An Active Process
It's crucial to understand that the RMP isn't passively achieved; it requires constant energy expenditure. The Na⁺/K⁺-ATPase, constantly working against the concentration gradients, is the primary mechanism ensuring the ionic imbalance necessary for the RMP. Without this pump's continuous activity, the concentration gradients would eventually dissipate, and the RMP would collapse.
Furthermore, other ion channels, though fewer in number at rest, contribute to fine-tuning the RMP. Slight changes in the activity of these channels, or alterations in extracellular ion concentrations, can subtly affect the resting membrane potential.
Consequences of Disruptions to the Resting Membrane Potential
The carefully maintained RMP is fundamental to neuronal function. Any significant disruption can have profound consequences. A depolarization, where the membrane potential becomes less negative (moves towards zero), is the first step in generating an action potential, the nerve impulse. Conversely, hyperpolarization, where the membrane potential becomes more negative, makes it harder to trigger an action potential.
Disruptions to the RMP can arise from various factors, including:
- Changes in extracellular ion concentrations: For example, elevated extracellular potassium levels can lead to depolarization, increasing neuronal excitability.
- Neurotransmitter action: Neurotransmitters released at synapses can bind to receptors on the neuronal membrane, opening or closing ion channels and altering the membrane potential.
- Drug effects: Certain drugs can directly or indirectly affect ion channels, influencing the RMP and neuronal excitability.
- Disease processes: Various diseases can disrupt ion channel function or alter ion concentrations, leading to abnormal neuronal activity.
Clinical Significance and Further Research
Understanding the intricacies of the RMP and its underlying ionic mechanisms is crucial in several fields of medicine and neuroscience. Disruptions to this delicate balance are implicated in numerous neurological and psychiatric disorders, including epilepsy, stroke, and various neurological diseases. Research continues to explore the subtle roles of different ion channels and transporters in health and disease, paving the way for novel therapeutic interventions.
For instance, research into specific ion channel subtypes could lead to the development of more targeted drugs to treat conditions like epilepsy, where abnormal neuronal excitability plays a major role. Likewise, a deeper understanding of how extracellular ion concentrations influence neuronal activity is vital for managing conditions like hyponatremia or hyperkalemia, where imbalances in sodium or potassium levels can severely affect neuronal function.
Furthermore, ongoing research is exploring the complexities of glial cell involvement in maintaining ionic homeostasis in the nervous system, recognizing that neurons do not operate in isolation. Glial cells, particularly astrocytes, play a crucial role in buffering extracellular potassium and glutamate, influencing neuronal excitability and synaptic transmission.
In conclusion, the seemingly simple concept of the resting membrane potential is a complex interplay of ionic concentrations, membrane permeability, and active transport mechanisms. This delicate balance is essential for neuronal function and the proper operation of the entire nervous system. Continued research into this fundamental process holds immense promise for advancing our understanding of neurological disorders and developing more effective therapies. The unequal distribution of ions, primarily Na⁺ and K⁺, coupled with the membrane's selective permeability, establishes the negative resting membrane potential – a crucial foundation for the transmission of nerve impulses and overall nervous system function.
Latest Posts
Latest Posts
-
In Photosynthesis Light Energy Is Converted To
Apr 11, 2025
-
Which Of The Following Is A Covalent Molecule
Apr 11, 2025
-
Actively Dividing Cells Can Be Found In
Apr 11, 2025
-
Venn Diagram Of Plant And Animal Cell
Apr 11, 2025
-
Advantages And Disadvantages Of Friction Force
Apr 11, 2025
Related Post
Thank you for visiting our website which covers about When A Nerve Fiber Is Polarized The Concentration Of . We hope the information provided has been useful to you. Feel free to contact us if you have any questions or need further assistance. See you next time and don't miss to bookmark.