What Is The Smallest Contractile Unit Of A Muscle
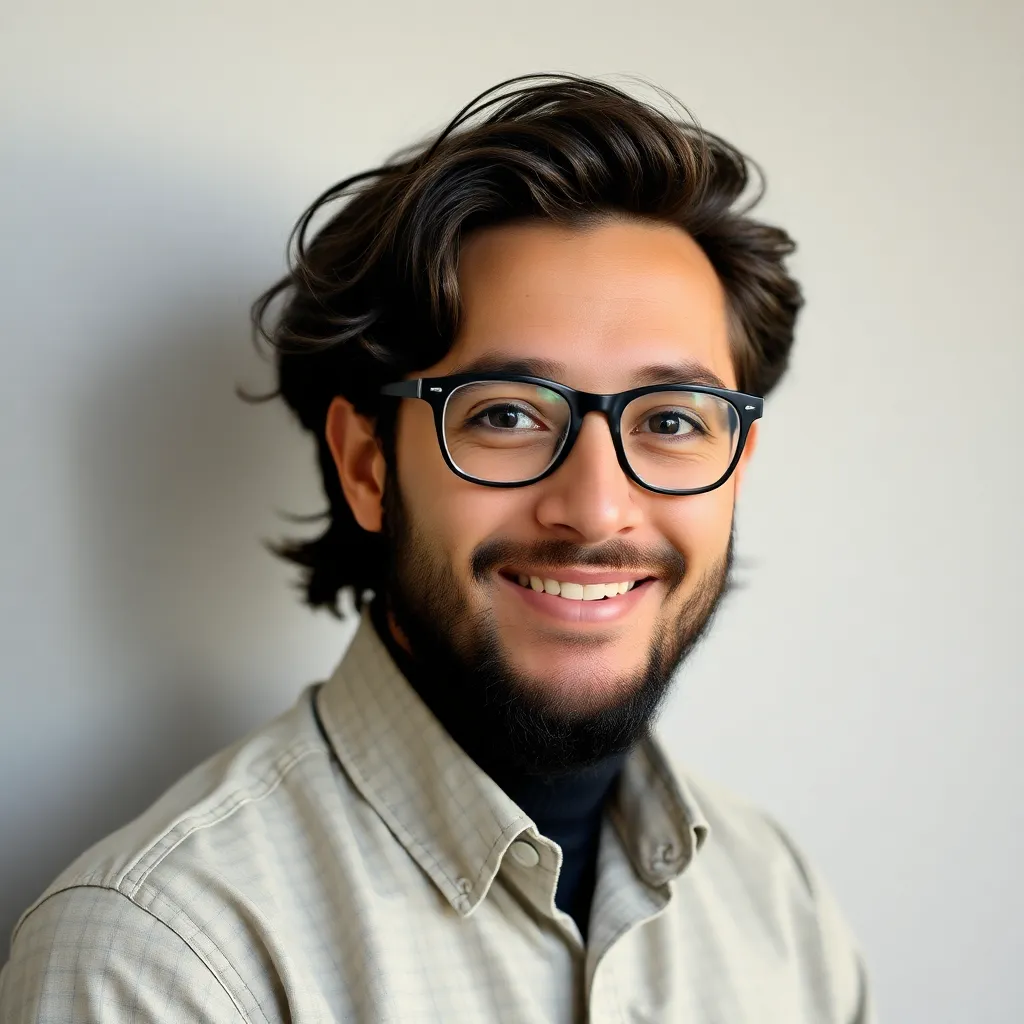
News Leon
Apr 16, 2025 · 7 min read

Table of Contents
What is the Smallest Contractile Unit of a Muscle?
The human body is a marvel of engineering, a complex system of interacting components working in perfect harmony. One of the most crucial systems is the muscular system, responsible for movement, posture, and a myriad of other vital functions. But at the heart of this system lies a fundamental unit of contraction: the sarcomere. This article delves deep into the fascinating world of sarcomeres, exploring their structure, function, and significance in muscle contraction. We will also touch upon the different types of muscle tissue and how sarcomere structure varies slightly to reflect the specialized functions of each.
Understanding the Sarcomere: The Basic Unit of Muscle Contraction
The sarcomere is the smallest functional unit of a muscle fiber. It's the fundamental repeating unit within a myofibril, which in turn are bundled together to form muscle fibers (muscle cells). Imagine a muscle fiber as a long, cylindrical cable; myofibrils are the individual strands within that cable, and sarcomeres are the tiny, repeating segments along each strand. This organized arrangement is crucial for efficient and coordinated muscle contraction.
Sarcomere Structure: A Detailed Look
The sarcomere's structure is highly organized and precisely arranged. Key components include:
-
Z-discs (Z-lines): These are dense, protein structures that serve as the boundaries of each sarcomere. They are composed primarily of α-actinin, a protein that anchors the thin filaments. The distance between two Z-discs defines the length of a sarcomere.
-
Thin filaments (actin filaments): These filaments extend from the Z-discs toward the center of the sarcomere. They are primarily composed of actin, a globular protein that polymerizes to form a double-stranded helix. Tropomyosin and troponin, regulatory proteins, are also integral parts of the thin filaments. Tropomyosin covers the myosin-binding sites on actin in a relaxed muscle, preventing contraction. Troponin acts as a calcium sensor, regulating tropomyosin's position and thus the interaction between actin and myosin.
-
Thick filaments (myosin filaments): Located in the center of the sarcomere, these filaments are composed of myosin, a motor protein with a head and tail region. The myosin heads have ATPase activity, allowing them to bind to actin and generate force. The thick filaments are arranged with their myosin heads projecting outwards, ready to interact with the thin filaments.
-
A-band (anisotropic band): This dark-staining band represents the entire length of the thick filaments. It includes the regions where thick and thin filaments overlap, as well as the central region containing only thick filaments.
-
I-band (isotropic band): This light-staining band contains only thin filaments and extends from the A-band of one sarcomere to the A-band of the next, being bisected by the Z-disc.
-
H-zone: This lighter area within the A-band represents the region where only thick filaments are present; thin filaments do not extend into this central region.
-
M-line: Located in the center of the H-zone, this dark line consists of proteins that hold the thick filaments in place.
The Sliding Filament Theory: How Sarcomeres Contract
Muscle contraction occurs through the sliding filament theory. This theory explains how the thin filaments slide past the thick filaments, causing the sarcomere to shorten. This shortening of individual sarcomeres, cumulatively, results in the contraction of the entire muscle fiber. The process is driven by the interaction between actin and myosin:
-
Calcium influx: A nerve impulse triggers the release of calcium ions (Ca²⁺) from the sarcoplasmic reticulum, a specialized organelle within muscle cells.
-
Troponin-tropomyosin complex shift: The released Ca²⁺ binds to troponin, causing a conformational change in the troponin-tropomyosin complex. This shift exposes the myosin-binding sites on the actin filaments.
-
Cross-bridge formation: The myosin heads, energized by ATP hydrolysis, bind to the exposed myosin-binding sites on actin, forming cross-bridges.
-
Power stroke: The myosin heads pivot, pulling the thin filaments towards the center of the sarcomere. This is the power stroke, generating the force of muscle contraction.
-
Cross-bridge detachment: ATP binds to the myosin head, causing it to detach from actin.
-
Myosin head re-energization: The ATP is hydrolyzed, re-energizing the myosin head, preparing it for another cycle of binding, pivoting, and detachment.
This cycle repeats continuously as long as Ca²⁺ is present and ATP is available, resulting in the sliding of thin filaments along thick filaments and subsequent sarcomere shortening. Relaxation occurs when Ca²⁺ is actively pumped back into the sarcoplasmic reticulum, causing the troponin-tropomyosin complex to return to its original position, blocking the myosin-binding sites on actin.
Sarcomere Variations in Different Muscle Types
While the basic sarcomere structure is common to all muscle types, there are subtle variations that reflect the specific functional demands of each muscle type:
Skeletal Muscle: Power and Precision
Skeletal muscle is responsible for voluntary movement and is characterized by its long, cylindrical fibers with multiple nuclei. Sarcomeres in skeletal muscle are highly organized, allowing for powerful and precise contractions. The arrangement of sarcomeres in parallel allows for significant force generation. Furthermore, the varying lengths and types of skeletal muscle fibers contribute to the diverse range of movements possible. Fast-twitch fibers contract rapidly but fatigue quickly, while slow-twitch fibers contract slowly but are resistant to fatigue.
Cardiac Muscle: Rhythmic Contractions
Cardiac muscle forms the heart and is responsible for rhythmic contractions that pump blood throughout the body. Cardiac muscle cells are branched and interconnected through intercalated discs, structures containing gap junctions that allow for rapid electrical signal transmission. Sarcomeres in cardiac muscle are similar to those in skeletal muscle, but they are shorter and more irregularly arranged. The interconnectedness of cardiac muscle cells ensures synchronized contractions. The presence of abundant mitochondria reflects the high energy demands of continuous heart contractions.
Smooth Muscle: Involuntary Control
Smooth muscle is found in the walls of internal organs and blood vessels, responsible for involuntary movements such as digestion and blood pressure regulation. Smooth muscle cells are spindle-shaped and lack the striated appearance of skeletal and cardiac muscle. Sarcomeres in smooth muscle are not as well-organized as in skeletal or cardiac muscle; the actin and myosin filaments are not arranged in regular arrays. Instead, they are attached to dense bodies within the cell, which act as anchoring points. This arrangement allows for sustained contractions over extended periods without fatigue.
Clinical Significance of Sarcomeres
Disruptions in sarcomere structure and function can lead to various muscle diseases:
-
Muscular dystrophies: A group of genetic disorders characterized by progressive muscle weakness and degeneration. These conditions often involve defects in proteins essential for sarcomere structure and function, leading to instability and muscle damage.
-
Cardiomyopathies: Diseases affecting the heart muscle, often resulting from abnormalities in sarcomere structure or function. These conditions can impair the heart's ability to pump blood effectively, leading to heart failure.
-
Inherited myopathies: A diverse group of disorders that affect muscle structure and function. Several of these myopathies are linked to specific defects in sarcomeric proteins.
Understanding sarcomere structure and function is crucial for the diagnosis and treatment of these and other muscle-related diseases. Research continues to uncover new insights into the intricate mechanisms governing sarcomere assembly, function, and regulation.
Conclusion: The Sarcomere's Crucial Role
The sarcomere, the smallest contractile unit of a muscle, is a marvel of biological engineering. Its highly organized structure and intricate molecular mechanisms enable efficient muscle contraction, powering movement, maintaining posture, and supporting vital functions throughout the body. Variations in sarcomere structure reflect the specialized functions of different muscle types, highlighting the adaptability and versatility of this fundamental unit. Furthermore, understanding the sarcomere is crucial for comprehending muscle diseases and developing potential treatments. The ongoing study of sarcomeres promises to unravel further mysteries of muscle function and improve our ability to address muscle-related disorders. The complexity and elegance of this tiny structure reinforce the remarkable intricacies of the human body and the power of nature's design.
Latest Posts
Latest Posts
-
Choose The Right Phrase To Complete The Sentence
Apr 19, 2025
-
Is Thymine A Purine Or Pyrimidine
Apr 19, 2025
-
An Element That Has Properties Of Both Metals And Nonmetals
Apr 19, 2025
-
Why Are Most Stomata On The Bottom Of The Leaf
Apr 19, 2025
-
Area Under An Acceleration Time Graph
Apr 19, 2025
Related Post
Thank you for visiting our website which covers about What Is The Smallest Contractile Unit Of A Muscle . We hope the information provided has been useful to you. Feel free to contact us if you have any questions or need further assistance. See you next time and don't miss to bookmark.