What Is The Basic Unit Of A Protein
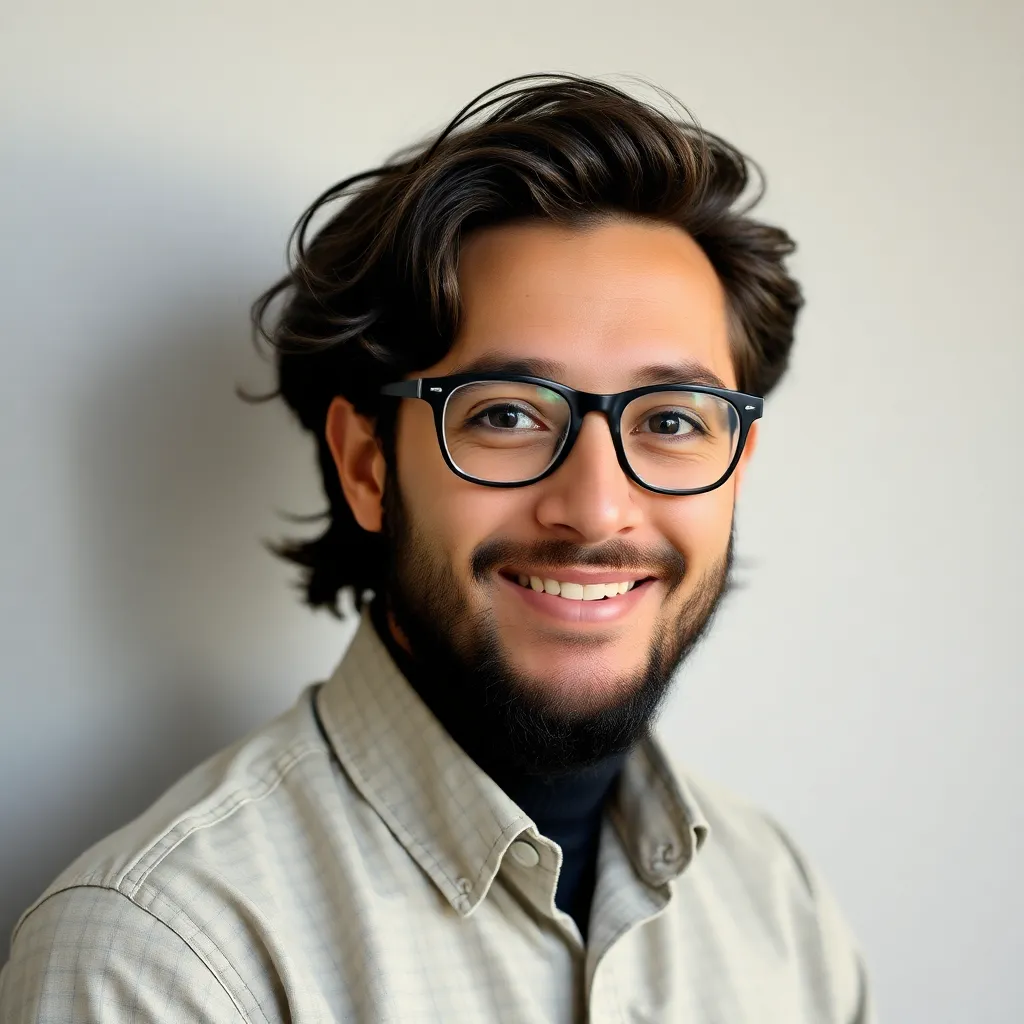
News Leon
Apr 17, 2025 · 6 min read

Table of Contents
What is the Basic Unit of a Protein?
Proteins are the workhorses of the cell, carrying out a vast array of functions essential for life. Understanding their structure is crucial to understanding their function. So, what exactly is the basic unit of a protein? The answer is simple, yet profound: amino acids.
Amino Acids: The Building Blocks of Proteins
Amino acids are organic molecules that serve as the fundamental monomers of proteins. Think of them as the individual letters that, when strung together in a specific sequence, form the words (proteins) of the genetic code. While there are hundreds of amino acids found in nature, only 20 standard amino acids are genetically encoded and used to build proteins in virtually all living organisms.
The General Structure of an Amino Acid
Each amino acid shares a common core structure:
- A central carbon atom (α-carbon): This carbon atom is bonded to four different chemical groups.
- An amino group (-NH₂): This group is basic and carries a positive charge at physiological pH.
- A carboxyl group (-COOH): This group is acidic and carries a negative charge at physiological pH.
- A hydrogen atom (-H): Simple, yet crucial to the overall structure.
- A variable side chain (R-group): This is the unique part of each amino acid, determining its chemical properties and influencing the protein's overall structure and function.
These R-groups are what differentiate the 20 standard amino acids. Some are nonpolar and hydrophobic (water-fearing), others are polar and hydrophilic (water-loving), and some even carry a charge at physiological pH. This diversity in R-group properties is essential for the formation of complex three-dimensional protein structures.
Classification of Amino Acids Based on R-Group Properties
The 20 standard amino acids can be broadly classified based on their R-group properties:
1. Nonpolar, Aliphatic Amino Acids: These amino acids have hydrocarbon side chains that are hydrophobic. Examples include: Glycine (Gly, G), Alanine (Ala, A), Valine (Val, V), Leucine (Leu, L), Isoleucine (Ile, I), and Methionine (Met, M).
2. Aromatic Amino Acids: These amino acids contain an aromatic ring in their side chains. They are generally hydrophobic but can participate in weak interactions with other molecules. Examples include: Phenylalanine (Phe, F), Tyrosine (Tyr, Y), and Tryptophan (Trp, W).
3. Polar, Uncharged Amino Acids: These amino acids have side chains that are polar but do not carry a net charge at physiological pH. They are hydrophilic and often participate in hydrogen bonding. Examples include: Serine (Ser, S), Threonine (Thr, T), Cysteine (Cys, C), Asparagine (Asn, N), and Glutamine (Gln, Q).
4. Positively Charged Amino Acids (Basic Amino Acids): These amino acids have side chains with a positive charge at physiological pH. They are hydrophilic and often interact with negatively charged molecules. Examples include: Lysine (Lys, K), Arginine (Arg, R), and Histidine (His, H).
5. Negatively Charged Amino Acids (Acidic Amino Acids): These amino acids have side chains with a negative charge at physiological pH. They are hydrophilic and often interact with positively charged molecules. Examples include: Aspartic acid (Asp, D) and Glutamic acid (Glu, E).
Peptide Bonds: Linking Amino Acids Together
Individual amino acids don't function as proteins on their own. They must be linked together to form a polypeptide chain. This linkage occurs through a peptide bond, a covalent bond formed between the carboxyl group of one amino acid and the amino group of another.
The formation of a peptide bond is a dehydration reaction, meaning a molecule of water is removed during the process. This bond is relatively strong and stable, contributing to the structural integrity of proteins.
From Amino Acid Sequence to Protein Structure: Levels of Protein Organization
The linear sequence of amino acids in a polypeptide chain determines its primary structure. However, this linear sequence then folds into more complex three-dimensional structures, leading to the higher levels of protein organization:
1. Primary Structure: The Amino Acid Sequence
The primary structure is simply the sequence of amino acids in a polypeptide chain. This sequence is dictated by the genetic code and is crucial because it determines all subsequent levels of protein structure. Even a single amino acid substitution can dramatically alter a protein's function, as seen in sickle cell anemia, where a single amino acid change in hemoglobin leads to a malfunctioning protein.
2. Secondary Structure: Local Folding Patterns
The primary structure begins to fold into regular, repeating patterns called secondary structures. These are stabilized by hydrogen bonds between the amino and carboxyl groups of the peptide backbone. The two most common secondary structures are:
- α-helices: A right-handed coiled structure stabilized by hydrogen bonds between every fourth amino acid.
- β-sheets: Extended polypeptide chains arranged side-by-side, forming a sheet-like structure. Hydrogen bonds stabilize the sheets between adjacent polypeptide chains.
Other secondary structures also exist, such as loops and turns, which connect α-helices and β-sheets.
3. Tertiary Structure: The Three-Dimensional Arrangement
The tertiary structure represents the overall three-dimensional arrangement of a polypeptide chain. It encompasses the spatial arrangement of all secondary structure elements and includes interactions between the R-groups of amino acids. These interactions include:
- Disulfide bonds: Covalent bonds formed between cysteine residues. These are strong and contribute significantly to protein stability.
- Hydrophobic interactions: Nonpolar R-groups cluster together in the protein's interior, away from the aqueous environment.
- Hydrogen bonds: Numerous hydrogen bonds occur between polar R-groups.
- Ionic bonds (salt bridges): Electrostatic interactions between oppositely charged R-groups.
The tertiary structure defines the protein's biological function.
4. Quaternary Structure: Multiple Polypeptide Chains
Some proteins consist of multiple polypeptide chains, each with its own tertiary structure. The arrangement of these individual subunits is called the quaternary structure. The subunits are held together by the same types of interactions as in tertiary structure. Examples of proteins with quaternary structure include hemoglobin and many enzymes.
Protein Function: A Diverse Array of Roles
The diverse functions of proteins arise directly from their unique three-dimensional structures. Proteins participate in virtually every cellular process, including:
- Catalysis: Enzymes are proteins that catalyze biochemical reactions.
- Structure: Proteins provide structural support to cells and tissues (e.g., collagen, keratin).
- Transport: Proteins transport molecules across cell membranes (e.g., membrane transporters) or in the bloodstream (e.g., hemoglobin).
- Movement: Proteins involved in muscle contraction (e.g., actin, myosin).
- Defense: Antibodies are proteins that protect the body from foreign invaders.
- Regulation: Proteins regulate gene expression and other cellular processes (e.g., transcription factors, hormones).
- Signaling: Proteins transmit signals within and between cells (e.g., receptor proteins).
The Importance of Protein Structure and Function in Health and Disease
The proper folding and function of proteins are essential for maintaining health. Errors in protein folding, or misfolding, can lead to a variety of diseases, including:
- Alzheimer's disease: Associated with the accumulation of misfolded amyloid-β proteins.
- Parkinson's disease: Linked to the aggregation of α-synuclein protein.
- Cystic fibrosis: Caused by a mutation in the CFTR protein, leading to misfolding and dysfunction.
- Prion diseases: Caused by misfolded prion proteins that induce other prion proteins to misfold, leading to neuronal damage.
Understanding the basic unit of a protein, the amino acid, and how it contributes to the intricate structure and function of proteins is crucial for advancing our knowledge in various fields, including medicine, biotechnology, and agriculture. Continued research into protein structure and function promises to yield further insights into the complexities of life and the development of novel therapeutic strategies.
Latest Posts
Latest Posts
-
Which Of The Following Is A Geometric Sequence
Apr 19, 2025
-
Why Is Aerobic Respiration More Efficient
Apr 19, 2025
-
Which Of The Following Statements Regarding Matter Is False
Apr 19, 2025
-
Is A Snake A Primary Consumer
Apr 19, 2025
-
An Economy Is Productively Efficient When
Apr 19, 2025
Related Post
Thank you for visiting our website which covers about What Is The Basic Unit Of A Protein . We hope the information provided has been useful to you. Feel free to contact us if you have any questions or need further assistance. See you next time and don't miss to bookmark.