What Happens When Gases Are Heated
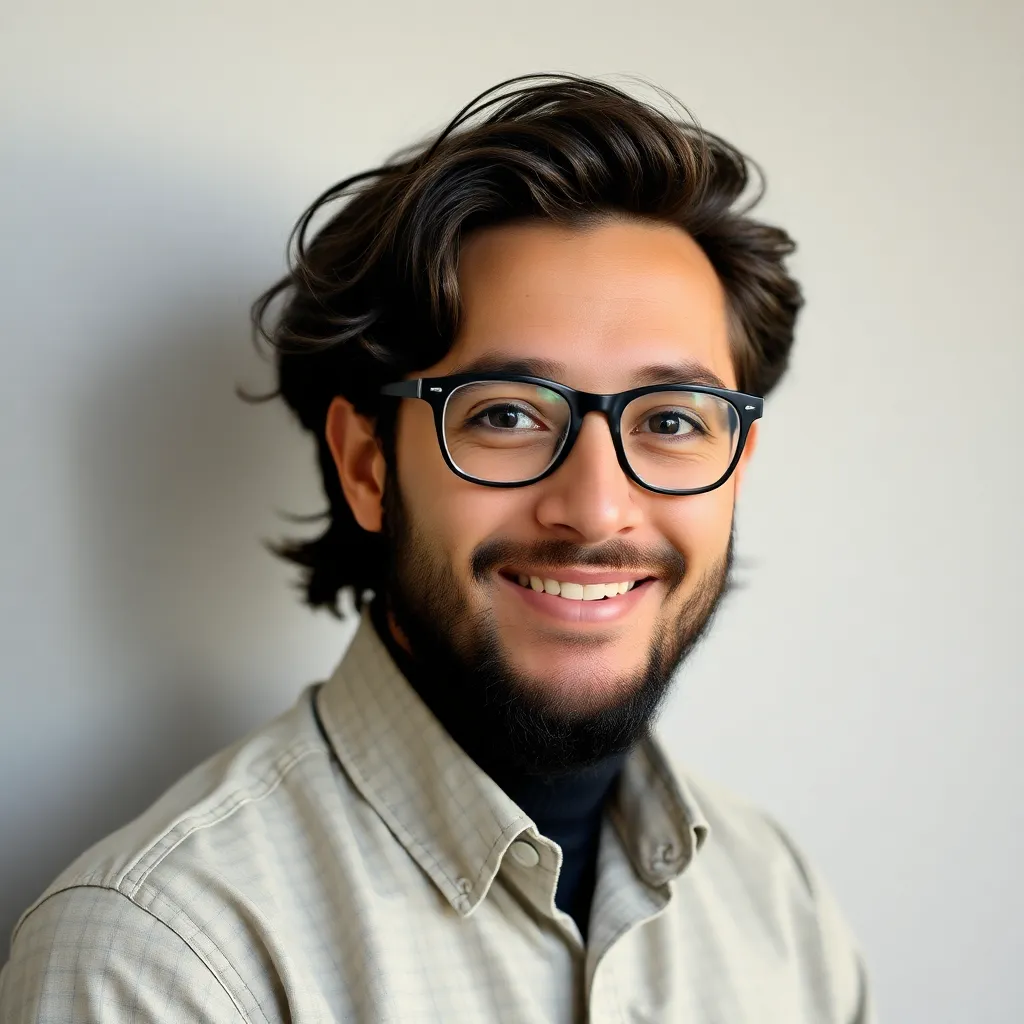
News Leon
Apr 09, 2025 · 5 min read

Table of Contents
What Happens When Gases Are Heated? A Deep Dive into Gas Behavior
Understanding how gases behave when heated is fundamental to numerous scientific fields, from meteorology and atmospheric science to engineering and industrial processes. This comprehensive guide explores the various effects of heating gases, examining the underlying physics and offering practical examples. We'll delve into concepts like thermal expansion, increased kinetic energy, changes in pressure and volume, and the implications for real-world applications.
The Kinetic Theory of Gases: The Foundation
Before examining the effects of heating gases, we need to understand the kinetic theory of gases. This model posits that gases consist of a vast number of tiny particles (atoms or molecules) in constant, random motion. These particles are far apart relative to their size, resulting in negligible intermolecular forces except during collisions.
The key characteristics of this model include:
- Constant, Random Motion: Gas particles are in perpetual motion, moving in straight lines until colliding with each other or the container walls.
- Negligible Intermolecular Forces: The attractive forces between gas particles are minimal compared to their kinetic energy, especially at higher temperatures and lower pressures.
- Elastic Collisions: Collisions between gas particles and the container walls are perfectly elastic, meaning no kinetic energy is lost.
- Average Kinetic Energy is Proportional to Temperature: This is the crucial link between temperature and gas behavior. Higher temperatures mean higher average kinetic energy of the particles.
Heating Gases: The Effects
Heating a gas leads to several interconnected effects, primarily stemming from the increase in the average kinetic energy of its constituent particles. Let's explore these in detail:
1. Increased Kinetic Energy and Velocity:
The most direct consequence of heating a gas is an increase in the average kinetic energy of its particles. This translates to a higher average velocity of these particles. The particles move faster, colliding more frequently and with greater force.
Example: Imagine heating air in a balloon. The air molecules gain kinetic energy, moving faster and colliding more forcefully with the balloon's inner surface, causing the balloon to expand.
2. Thermal Expansion:
As the kinetic energy of gas particles increases, they collide more forcefully with the container walls. This increased pressure pushes against the walls, causing the gas to expand if the container is flexible. If the container is rigid, the pressure inside will increase.
Charles's Law: This law states that at constant pressure, the volume of a gas is directly proportional to its absolute temperature. Mathematically, it's represented as V/T = k, where V is volume, T is absolute temperature (in Kelvin), and k is a constant.
Example: A hot air balloon rises because the heated air inside expands, becoming less dense than the surrounding cooler air. This density difference creates buoyant force, lifting the balloon.
3. Increased Pressure:
If the volume of a gas is kept constant while it's heated, the increase in kinetic energy leads to more frequent and forceful collisions with the container walls. This results in a higher pressure.
Gay-Lussac's Law: This law states that at constant volume, the pressure of a gas is directly proportional to its absolute temperature. Mathematically, it's expressed as P/T = k, where P is pressure, T is absolute temperature (in Kelvin), and k is a constant.
Example: A pressure cooker utilizes this principle. Heating the food inside increases the pressure, raising the boiling point of water and allowing food to cook faster at higher temperatures.
4. Changes in Density:
Heating a gas at constant pressure causes it to expand, thereby decreasing its density. Conversely, heating a gas at constant volume increases its pressure but doesn't change its density.
Example: A hot air balloon's heated air has lower density than the surrounding cooler air, enabling it to float.
5. Changes in Molecular Behavior:
At higher temperatures, some gases may undergo changes in their molecular structure. For instance, diatomic molecules like oxygen (O2) may dissociate into individual atoms at extremely high temperatures. This alters the gas's properties significantly.
6. Real vs. Ideal Gases: Deviations from Ideal Behavior
The kinetic theory describes ideal gases, which assume perfectly elastic collisions and negligible intermolecular forces. Real gases, however, deviate from ideal behavior, particularly at high pressures and low temperatures where intermolecular forces become significant. At high temperatures and low pressures, however, most gases exhibit behaviour very close to ideal.
Compressibility Factor: This factor (Z) quantifies the deviation of a real gas from ideal behavior. Z = PV/RT, where P is pressure, V is volume, R is the ideal gas constant, and T is temperature. For an ideal gas, Z = 1. Deviations from 1 indicate non-ideal behavior.
Van der Waals Equation: This equation accounts for intermolecular forces and the finite volume of gas particles, providing a more accurate description of real gas behavior.
Practical Applications and Real-World Examples:
The effects of heating gases are fundamental to numerous technologies and natural phenomena:
- Internal Combustion Engines: Heating a fuel-air mixture causes rapid expansion, generating power to propel vehicles.
- Power Generation: Heating water to produce steam drives turbines in power plants, generating electricity.
- Meteorology: Understanding how air temperature affects pressure and density is crucial for weather forecasting.
- Aerospace Engineering: Designing rockets and aircraft requires detailed knowledge of gas behavior at varying altitudes and temperatures.
- Chemical Processes: Many industrial chemical processes rely on heating gases to drive reactions or separations.
- Refrigeration: Refrigerants utilize the principles of gas expansion and compression to transfer heat.
- Hot Air Balloons: The principle of buoyancy driven by heated air is a classic example.
Conclusion:
Heating a gas leads to a series of interconnected changes, primarily due to the increase in the average kinetic energy of its constituent particles. This results in thermal expansion, increased pressure, changes in density, and potential alterations in molecular behavior. These effects are crucial across various scientific disciplines and have far-reaching applications in numerous technologies and natural phenomena. Understanding these principles is vital for advancements in diverse fields, ranging from engineering and manufacturing to atmospheric science and climate modeling. Further exploration of specific gas behaviors under different conditions can provide even greater insights into this fundamental aspect of physics and chemistry. The complex interplay of temperature, pressure, and volume in heated gases opens up possibilities for further scientific investigation and technological innovation. Ongoing research continues to refine our understanding of gas behavior and its applications in various technological processes.
Latest Posts
Latest Posts
-
The Path Followed By A Projectile Is Called
Apr 18, 2025
-
Dissolution Of Urea In Water Equation
Apr 18, 2025
-
Any Place Where Two Bones Meet
Apr 18, 2025
-
A Scientific Name Contains Information About Its
Apr 18, 2025
-
The Most Widespread And Abundant Tissue In The Body Is
Apr 18, 2025
Related Post
Thank you for visiting our website which covers about What Happens When Gases Are Heated . We hope the information provided has been useful to you. Feel free to contact us if you have any questions or need further assistance. See you next time and don't miss to bookmark.