What Are Three Parts Of Atp Molecule
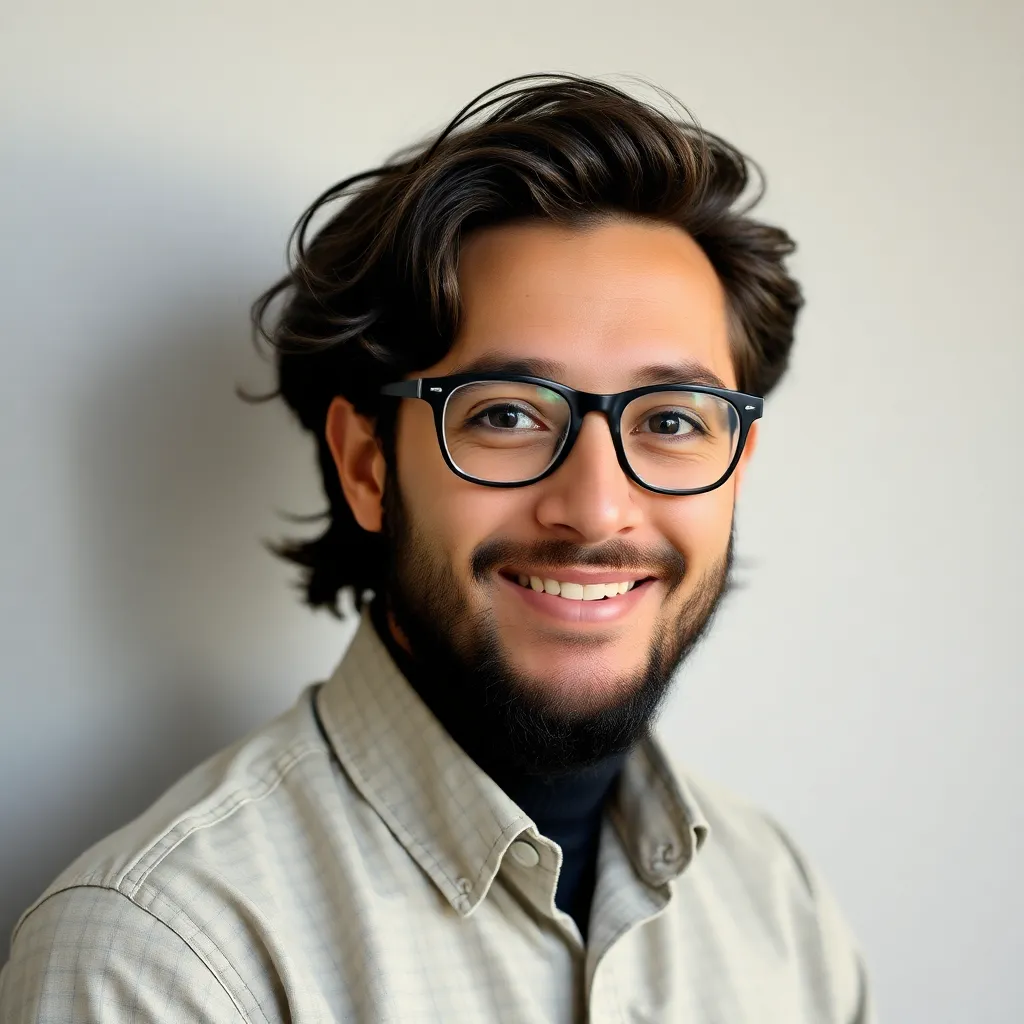
News Leon
Apr 18, 2025 · 6 min read

Table of Contents
What are the Three Parts of an ATP Molecule? Unlocking the Energy Currency of Life
Adenosine triphosphate (ATP) is the fundamental energy currency of all living cells. It powers countless cellular processes, from muscle contraction and protein synthesis to nerve impulse transmission and active transport. Understanding the structure of ATP is key to grasping its remarkable ability to store and release energy. This article delves deep into the three components of the ATP molecule, explaining their individual roles and how their arrangement facilitates ATP's crucial function. We will explore its structure, its role in cellular respiration, and its significance in maintaining life itself. By understanding the intricacies of the ATP molecule, we can better appreciate the complexity and elegance of biological systems.
The Three Building Blocks: Adenine, Ribose, and Phosphate Groups
The ATP molecule is composed of three distinct parts:
-
Adenine: A nitrogenous base, a crucial component of DNA and RNA. Its planar structure contributes to the overall stability of the ATP molecule. Specifically, adenine's aromatic ring system allows for efficient energy transfer through resonance stabilization.
-
Ribose: A five-carbon sugar (pentose) that forms the backbone of the ATP molecule. It's a ribose sugar, meaning it possesses a hydroxyl group (-OH) on the 2' carbon atom, differentiating it from deoxyribose found in DNA. This hydroxyl group plays a role in the molecule's reactivity and solubility in water. The ribose sugar acts as the linking structure between adenine and the phosphate groups. The specific arrangement of the ribose atoms determines the three-dimensional conformation of the ATP molecule, which is vital for its interaction with enzymes.
-
Three Phosphate Groups: This is arguably the most crucial component of ATP. These three phosphate groups, denoted as alpha (α), beta (β), and gamma (γ), are linked together by high-energy phosphoanhydride bonds. These bonds are the key to ATP's energy storage capacity. The high energy is stored within these bonds due to the negative charges repelling each other; this repulsion creates an unstable situation. The energy is released when these bonds are broken through hydrolysis.
Understanding High-Energy Phosphoanhydride Bonds
The phosphoanhydride bonds connecting the phosphate groups are not ordinary chemical bonds. They are high-energy bonds. This high energy isn't stored in the bonds themselves, but rather is a consequence of the electrostatic repulsion between the negatively charged phosphate groups. The proximity of these negatively charged groups creates a state of high potential energy. This is analogous to a tightly coiled spring; the energy is stored in the configuration.
When a phosphate group is cleaved off through hydrolysis (a reaction with water), this repulsion is relieved, releasing a substantial amount of energy that can then be used to drive other cellular processes. This process typically involves the enzyme ATPase, which catalyzes the hydrolysis of ATP to ADP (adenosine diphosphate) and inorganic phosphate (Pi).
The equation for this hydrolysis is:
ATP + H₂O → ADP + Pi + Energy
This released energy is not free-floating; it's immediately coupled to an endergonic reaction (a reaction requiring energy input) to drive it forward. This coupling is a fundamental principle of energy transfer in biological systems.
The Role of ATP in Cellular Respiration: From Food to Energy
ATP is the final product of cellular respiration, the process by which cells break down glucose and other energy-rich molecules to produce usable energy. Cellular respiration involves a series of intricate biochemical reactions, which can be broadly categorized into three main stages:
-
Glycolysis: This occurs in the cytoplasm and breaks down glucose into pyruvate. While glycolysis produces a small amount of ATP directly, its primary role is to generate NADH, a crucial electron carrier, and pyruvate, which enters the mitochondria for further energy extraction.
-
Krebs Cycle (Citric Acid Cycle): Within the mitochondria, pyruvate is further oxidized, releasing carbon dioxide and generating more electron carriers (NADH and FADH2), and a small amount of ATP.
-
Oxidative Phosphorylation (Electron Transport Chain and Chemiosmosis): This is the stage where the majority of ATP is produced. The electron carriers generated in glycolysis and the Krebs cycle donate their electrons to the electron transport chain, embedded in the inner mitochondrial membrane. This electron flow drives the pumping of protons (H+) across the membrane, creating a proton gradient. This gradient then drives ATP synthase, an enzyme that synthesizes ATP from ADP and Pi. This process is called chemiosmosis.
The entire process of cellular respiration is incredibly efficient at converting the chemical energy stored in glucose into the readily usable energy stored in ATP. The ATP molecules produced then act as energy carriers, transferring this energy to various cellular processes as needed.
ATP and Other Nucleotides: A Family Resemblance
ATP belongs to a family of molecules known as nucleotides. Other important nucleotides include:
-
ADP (Adenosine Diphosphate): This molecule is formed when a phosphate group is removed from ATP. It can be re-phosphorylated to form ATP through processes like oxidative phosphorylation.
-
AMP (Adenosine Monophosphate): This is formed when a second phosphate group is removed from ADP. It has a lower energy content compared to ADP and ATP.
-
GTP (Guanosine Triphosphate): Similar in structure to ATP but with guanine instead of adenine. GTP also plays a crucial role in energy transfer in certain cellular processes, particularly protein synthesis.
-
cAMP (Cyclic Adenosine Monophosphate): A crucial second messenger molecule involved in cellular signaling pathways. While it doesn't directly store energy like ATP, it plays a vital role in transmitting signals within the cell.
These molecules highlight the versatility of nucleotide structures and their varied roles in cellular function.
Beyond Energy Transfer: Other Roles of ATP
While its primary function is energy transfer, ATP plays numerous other essential roles within the cell:
-
Enzyme Regulation: ATP can directly regulate the activity of many enzymes, often by acting as an allosteric effector, binding to a site other than the active site and influencing enzyme conformation and activity.
-
Signal Transduction: ATP is involved in various signaling pathways, often acting as a substrate for kinases, enzymes that phosphorylate other proteins, thereby altering their activity.
-
DNA and RNA Synthesis: ATP provides the energy necessary for the synthesis of DNA and RNA, crucial processes for cell replication and gene expression.
-
Active Transport: ATP powers active transport mechanisms, allowing cells to move molecules against their concentration gradients, a process essential for maintaining cellular homeostasis.
ATP and Disease: The Consequences of Dysfunction
Disruptions in ATP production or utilization can lead to various diseases and disorders. Mitochondrial diseases, for instance, often stem from defects in the mitochondrial electron transport chain, reducing ATP production and impacting energy-dependent cellular functions. These diseases can manifest in a wide range of symptoms, depending on the specific affected tissues.
Similarly, disruptions in ATP-dependent processes can also be implicated in several other pathologies, including muscle disorders, neurological diseases, and various metabolic syndromes. Further research into the intricate roles of ATP continues to reveal new insights into disease mechanisms and potential therapeutic strategies.
Conclusion: The Indispensable ATP Molecule
The adenosine triphosphate molecule, with its three integral components—adenine, ribose, and three phosphate groups—stands as a cornerstone of life. Its ability to store and release energy efficiently drives countless cellular processes, making it the undisputed energy currency of all living organisms. Understanding its structure, function, and the implications of its dysfunction is crucial for advancing our knowledge of biological systems and developing novel therapeutic approaches to various diseases. The exploration of ATP continues to be a vital area of research, constantly unveiling new facets of this remarkable molecule and its profound impact on life itself. Further research promises to shed even more light on its multifaceted roles and the intricacies of its interactions within the complex tapestry of cellular life.
Latest Posts
Latest Posts
-
Why Circle Is Not A Polygon
Apr 19, 2025
-
A Solution With A Ph Value Less Than 7 Is
Apr 19, 2025
-
Is Mixing Water And Sugar A Chemical Change
Apr 19, 2025
-
A Group Of People Are Called
Apr 19, 2025
-
What Is The Major Intracellular Cation
Apr 19, 2025
Related Post
Thank you for visiting our website which covers about What Are Three Parts Of Atp Molecule . We hope the information provided has been useful to you. Feel free to contact us if you have any questions or need further assistance. See you next time and don't miss to bookmark.