The Science Of Heredity Is Called
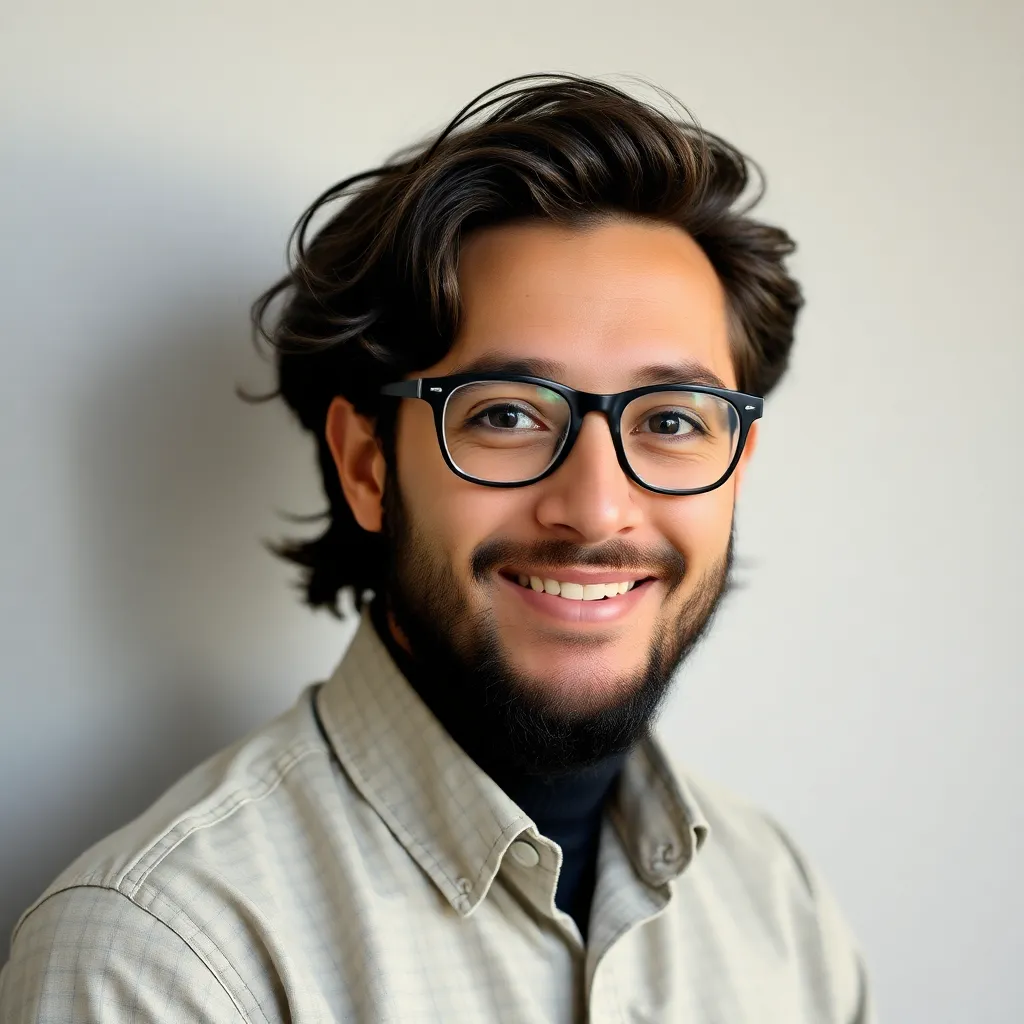
News Leon
Mar 12, 2025 · 8 min read

Table of Contents
The Science of Heredity: Exploring the Fascinating World of Genetics
The science of heredity is called genetics. It's a field brimming with intricate details, exploring how characteristics are passed down from one generation to the next. From the subtle nuances of eye color to the complex interplay of genes determining disease susceptibility, genetics unravels the fundamental blueprint of life. This comprehensive exploration delves into the core principles of genetics, exploring its history, key concepts, applications, and future implications.
A Brief History of Genetics: From Mendel's Peas to the Human Genome Project
The formal study of genetics began with Gregor Mendel's groundbreaking experiments in the mid-1800s. Mendel, an Austrian monk, meticulously documented the inheritance patterns of traits in pea plants. His work, published in 1866, laid the foundation for understanding the basic principles of heredity, including the concepts of dominant and recessive alleles, homozygous and heterozygous genotypes, and the segregation and independent assortment of genes. However, his contributions remained largely unrecognized until the early 20th century.
The rediscovery of Mendel's work in the early 1900s sparked a revolution in biological sciences. Scientists began to unravel the mechanisms underlying inheritance, leading to the development of the chromosome theory of inheritance, which posited that genes are located on chromosomes within the cell nucleus. This understanding paved the way for significant advancements in genetics, including the discovery of DNA as the carrier of genetic information.
The latter half of the 20th century witnessed an explosion of discoveries in molecular biology, leading to the elucidation of the structure of DNA by Watson and Crick in 1953. This landmark achievement revolutionized genetics, providing a molecular basis for understanding how genetic information is stored, replicated, and expressed. The development of techniques like polymerase chain reaction (PCR) further amplified the ability to study and manipulate DNA, opening up new avenues of research.
The culmination of decades of research culminated in the completion of the Human Genome Project in 2003. This monumental effort mapped the entire human genome, providing a detailed inventory of all human genes and their locations on chromosomes. This achievement marked a pivotal moment in the history of genetics, paving the way for advancements in personalized medicine, disease diagnostics, and genetic engineering.
Core Concepts in Genetics: Understanding the Language of Life
Several fundamental concepts underpin the science of heredity. Understanding these concepts is crucial for grasping the complexities of genetic inheritance and its applications.
Genes, Alleles, and Genotypes: The Building Blocks of Inheritance
A gene is a specific sequence of DNA that codes for a particular trait or characteristic. Different versions of a gene are called alleles. For example, a gene for eye color might have alleles for brown eyes and blue eyes. An individual inherits two alleles for each gene, one from each parent. The combination of alleles an individual possesses for a particular gene is called their genotype.
Phenotype: The Observable Expression of Genes
The observable characteristics of an individual, resulting from the interaction of their genotype and the environment, is called their phenotype. For instance, an individual with two alleles for brown eyes (homozygous dominant) will have brown eyes, while an individual with one allele for brown eyes and one for blue eyes (heterozygous) might have brown eyes (if brown is dominant) or a different eye color altogether depending on the specific alleles and their interactions.
Dominant and Recessive Alleles: Determining Trait Expression
Alleles can be classified as dominant or recessive. A dominant allele will express its phenotype even if only one copy is present (heterozygous genotype). A recessive allele will only express its phenotype if two copies are present (homozygous recessive genotype). The interaction between dominant and recessive alleles determines the phenotype of an individual.
Homozygous and Heterozygous Genotypes: The Allelic Combinations
An individual is homozygous for a gene if they have two identical alleles (e.g., two alleles for brown eyes). They are heterozygous if they have two different alleles (e.g., one allele for brown eyes and one for blue eyes).
Segregation and Independent Assortment: The Laws of Mendelian Inheritance
Mendel's laws of inheritance describe how alleles segregate during gamete formation (meiosis) and how different genes assort independently. The law of segregation states that each parent contributes one allele for each gene to their offspring. The law of independent assortment states that different genes are inherited independently of one another, meaning the inheritance of one gene does not influence the inheritance of another. These laws provide a basic framework for understanding how genetic variation arises and is transmitted across generations.
Genetic Linkage and Crossing Over: Exceptions to Independent Assortment
While Mendel's law of independent assortment holds true for many genes, some genes located close together on the same chromosome tend to be inherited together. This phenomenon is known as genetic linkage. However, during meiosis, a process called crossing over can occur, where homologous chromosomes exchange segments of DNA, resulting in recombination of alleles and breaking up linked genes. Crossing over contributes to genetic diversity and shuffles the genetic material between chromosomes.
Beyond Mendelian Genetics: Exploring Complex Inheritance Patterns
While Mendel's laws provide a foundation for understanding heredity, many traits do not follow simple dominant-recessive inheritance patterns. Complex inheritance involves multiple genes interacting with each other and the environment to influence a single trait. Examples include height, weight, and susceptibility to many diseases.
Polygenic Inheritance: The Influence of Multiple Genes
Polygenic inheritance involves traits determined by the combined effect of multiple genes. These traits often exhibit continuous variation, meaning they can take on a wide range of values (e.g., height). The phenotype of a polygenic trait is influenced by the cumulative effect of many genes, each contributing a small effect.
Pleiotropy: One Gene, Multiple Effects
Pleiotropy refers to the phenomenon where a single gene influences multiple seemingly unrelated traits. This occurs because the protein product of a gene may have multiple functions or affect different pathways within the cell. A classic example is phenylketonuria (PKU), a genetic disorder affecting metabolism, which leads to multiple symptoms including intellectual disability, seizures, and skin abnormalities.
Epigenetics: Environmental Influence on Gene Expression
Epigenetics is the study of heritable changes in gene expression that do not involve alterations to the underlying DNA sequence. Environmental factors such as diet, stress, and exposure to toxins can influence epigenetic modifications, affecting gene expression and potentially leading to changes in phenotype. Epigenetic modifications can be passed on to subsequent generations, highlighting the intricate interplay between genes and environment.
Applications of Genetics: Revolutionizing Medicine and Beyond
Genetics has had a profound impact on various fields, revolutionizing our understanding of disease, agriculture, and evolution.
Medical Genetics: Diagnosing and Treating Genetic Disorders
Medical genetics plays a crucial role in diagnosing and managing genetic disorders. Genetic testing can identify mutations responsible for inherited diseases, enabling early diagnosis, genetic counseling, and personalized treatment approaches. Advances in gene therapy hold promise for treating genetic disorders by correcting or replacing faulty genes.
Forensic Genetics: Utilizing DNA for Identification and Investigation
Forensic genetics utilizes DNA analysis to identify individuals, establish paternity, and investigate crimes. DNA profiling techniques have revolutionized forensic science, providing powerful tools for solving criminal cases and identifying missing persons.
Agricultural Genetics: Improving Crop Yields and Disease Resistance
Agricultural genetics aims to improve crop yields, disease resistance, and nutritional value. Techniques such as genetic modification (GM) and marker-assisted selection are used to develop crops with enhanced characteristics, contributing to food security and sustainable agriculture.
Evolutionary Genetics: Understanding the History of Life
Evolutionary genetics examines the role of genetic variation in driving evolution. By analyzing DNA sequences and studying genetic variation within and between populations, scientists can infer evolutionary relationships, track the spread of genes, and understand the mechanisms of adaptation.
The Future of Genetics: Exploring Uncharted Territories
The field of genetics continues to evolve rapidly, with new technologies and discoveries pushing the boundaries of our understanding. Several exciting areas of research hold promise for the future:
CRISPR-Cas9 Gene Editing: Precise Manipulation of the Genome
CRISPR-Cas9 technology has revolutionized gene editing, allowing scientists to precisely target and modify DNA sequences with unprecedented accuracy. This technology holds tremendous potential for treating genetic diseases, developing new therapies, and advancing basic research.
Personalized Medicine: Tailoring Treatment to Individual Genomes
Personalized medicine aims to tailor medical treatment to individual patients based on their unique genetic makeup. By analyzing an individual's genome, doctors can predict disease risk, select the most effective treatments, and minimize adverse drug reactions, ushering in an era of personalized healthcare.
Synthetic Biology: Designing and Engineering Biological Systems
Synthetic biology seeks to design and engineer new biological systems with novel functions. This emerging field holds potential for developing new drugs, biofuels, and materials, as well as for advancing our understanding of fundamental biological processes.
Genomics and Big Data: Analyzing Vast Datasets to Uncover Insights
The accumulation of vast genomic datasets presents both challenges and opportunities for researchers. Developing advanced computational tools and bioinformatics approaches to analyze these datasets will be crucial for uncovering new insights into human biology, disease, and evolution.
In conclusion, the science of heredity, genetics, is a dynamic and ever-evolving field. From Mendel's foundational work to the cutting-edge technologies of today, genetics has transformed our understanding of life itself, offering profound implications for medicine, agriculture, and our very understanding of the natural world. The future of genetics promises even more groundbreaking discoveries, further revolutionizing how we approach health, agriculture, and the very fabric of life.
Latest Posts
Related Post
Thank you for visiting our website which covers about The Science Of Heredity Is Called . We hope the information provided has been useful to you. Feel free to contact us if you have any questions or need further assistance. See you next time and don't miss to bookmark.