The Reactivity Of An Atom Depends On The Number Of
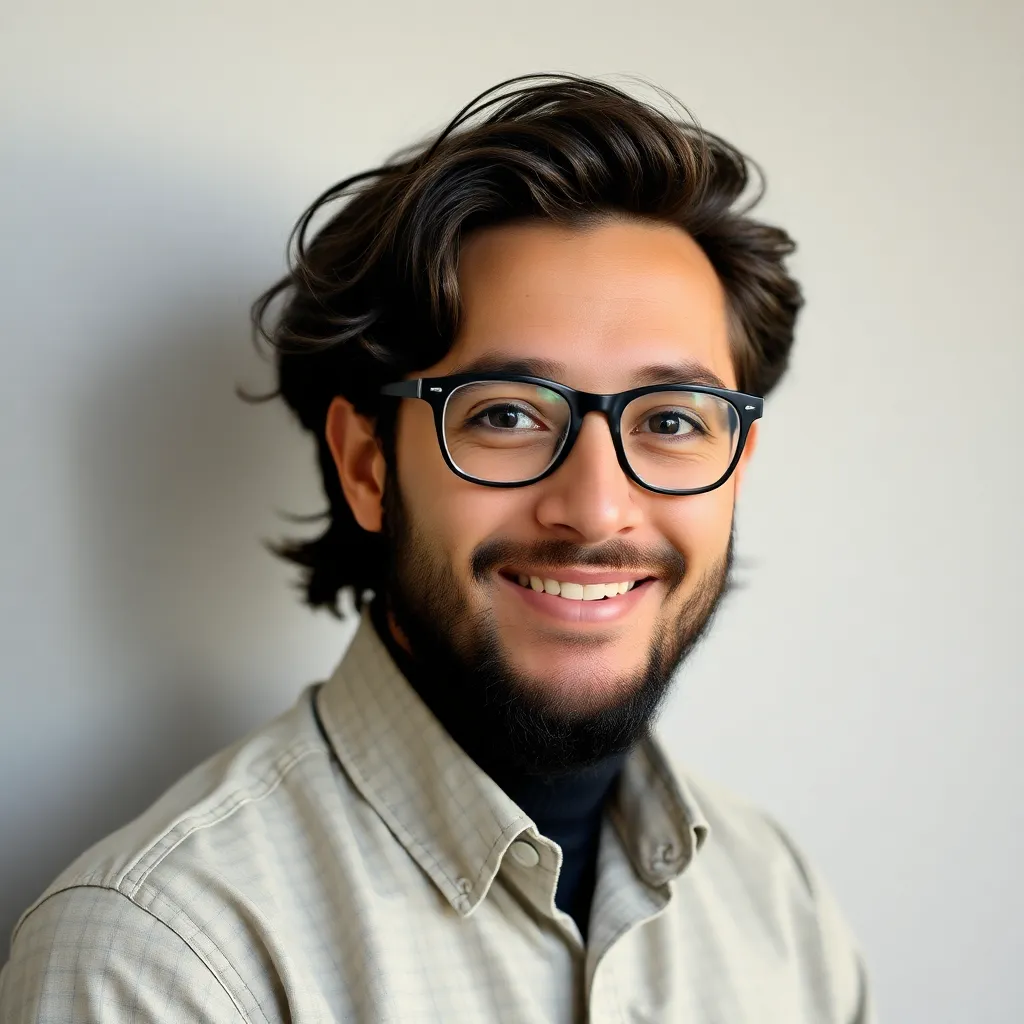
News Leon
Apr 26, 2025 · 6 min read

Table of Contents
The Reactivity of an Atom Depends on the Number of: Valence Electrons and Beyond
The reactivity of an atom, its inherent tendency to participate in chemical reactions, is fundamentally determined by the number of valence electrons it possesses. This seemingly simple statement belies a complex interplay of factors that govern the behavior of atoms in the macroscopic world. While the number of valence electrons is paramount, other properties, such as electronegativity and effective nuclear charge, significantly influence an atom's reactivity. This article will delve deep into the relationship between an atom's electron configuration, particularly its valence electrons, and its resultant reactivity, exploring the nuances that shape chemical behavior.
Valence Electrons: The Key Players in Reactivity
Atoms strive for stability, a state characterized by a full outermost electron shell. This is often achieved by gaining, losing, or sharing electrons to attain a noble gas configuration, a state with eight valence electrons (the octet rule, with some exceptions for lighter elements). The number of valence electrons an atom possesses directly dictates how it will achieve this stability, thereby determining its reactivity.
Groups in the Periodic Table and Reactivity
The periodic table provides a valuable framework for understanding reactivity. Elements within the same group (vertical column) share the same number of valence electrons, resulting in similar chemical behaviors.
-
Group 1 (Alkali Metals): These elements have one valence electron, readily lost to form +1 ions. This ease of electron loss makes them highly reactive, readily reacting with water and other substances. For example, Sodium (Na) violently reacts with water, producing hydrogen gas and sodium hydroxide.
-
Group 2 (Alkaline Earth Metals): Possessing two valence electrons, these metals are also reactive, albeit less so than alkali metals. They tend to lose two electrons to form +2 ions. Magnesium (Mg), for instance, burns brightly in air, reacting with oxygen to form magnesium oxide.
-
Group 17 (Halogens): With seven valence electrons, halogens are highly reactive nonmetals. They readily gain one electron to achieve a stable octet, forming -1 ions. Chlorine (Cl), for example, readily reacts with sodium (Na) to form sodium chloride (NaCl), common table salt.
-
Group 18 (Noble Gases): These elements have a full valence shell (eight electrons, except for helium with two), making them extremely unreactive. Their stability prevents them from readily participating in chemical reactions. This inertness makes them useful in applications requiring non-reactivity, such as lighting and shielding.
Beyond the Octet Rule: Exceptions and Nuances
While the octet rule provides a useful framework, it's not without exceptions. Some elements, particularly those in the third and subsequent periods, can accommodate more than eight electrons in their valence shell, forming expanded octets. This is often observed in compounds of phosphorus and sulfur, where they can form more than four bonds. Transition metals also exhibit complex reactivity patterns, often forming multiple oxidation states due to the involvement of d-electrons in bonding.
Other Factors Influencing Reactivity
Beyond valence electrons, several other atomic properties significantly influence an atom's reactivity:
Electronegativity: The Tug-of-War for Electrons
Electronegativity measures an atom's ability to attract electrons in a chemical bond. High electronegativity implies a strong pull on shared electrons, resulting in polar bonds and potentially ionic bonds. Fluorine (F), the most electronegative element, readily attracts electrons from other atoms, leading to its high reactivity. Conversely, elements with low electronegativity tend to lose electrons easily, contributing to their reactivity as well. The difference in electronegativity between atoms involved in a bond determines the polarity and the nature of the bond itself (ionic, covalent, polar covalent).
Effective Nuclear Charge: The Net Attraction
Effective nuclear charge represents the net positive charge experienced by an electron in an atom. It's the difference between the actual nuclear charge and the shielding effect of inner electrons. A higher effective nuclear charge leads to a stronger attraction for valence electrons, influencing the atom's ionization energy (energy required to remove an electron) and its tendency to form bonds. Elements with high effective nuclear charge tend to be less reactive as their valence electrons are tightly held.
Atomic Radius: Size Matters
Atomic radius, the size of an atom, also affects reactivity. Larger atoms have valence electrons farther from the nucleus, experiencing weaker attraction. Consequently, these valence electrons are more easily lost or shared, resulting in higher reactivity. Smaller atoms with tightly held valence electrons tend to be less reactive.
Ionization Energy and Electron Affinity: Energy Considerations
Ionization energy quantifies the energy needed to remove an electron from an atom. Low ionization energy signifies that an atom readily loses electrons, increasing its reactivity. Electron affinity, on the other hand, measures the energy change when an atom gains an electron. High electron affinity indicates that an atom readily gains electrons, also increasing its reactivity. These energies are directly linked to an atom's position within the periodic table and its electron configuration.
Predicting Reactivity: A Multifaceted Approach
Predicting the reactivity of an atom requires a holistic approach, considering the interplay of valence electrons, electronegativity, effective nuclear charge, atomic radius, ionization energy, and electron affinity. The periodic table provides a framework for understanding trends, but individual element behavior may deviate slightly due to the complex interplay of these factors.
For instance, while alkali metals are generally highly reactive due to their single valence electron, lithium (Li), the smallest alkali metal, shows some unique reactivity patterns compared to its heavier counterparts. Its small size leads to a higher charge density, influencing its interactions with other atoms.
Similarly, transition metals exhibit complex reactivity, forming multiple oxidation states due to the involvement of d-electrons. This complexity makes their reactivity less straightforward compared to main group elements.
Applications and Implications
Understanding atomic reactivity is crucial across various scientific fields. This knowledge is fundamental to:
-
Chemical Synthesis: Predicting and controlling chemical reactions requires a thorough understanding of the reactivity of the atoms involved. This is essential in developing new materials and drugs.
-
Materials Science: The properties of materials, such as strength, conductivity, and reactivity, are fundamentally linked to the reactivity of their constituent atoms. This understanding is vital in developing new materials with specific properties.
-
Environmental Science: Understanding the reactivity of elements is crucial for assessing environmental impact. The reactivity of pollutants, for instance, dictates their persistence and potential harm to the environment.
-
Biological Systems: Biological processes rely on the precise interplay of atomic reactivity. Enzymes, for instance, facilitate reactions by precisely manipulating the reactivity of molecules.
Conclusion
The reactivity of an atom is a multifaceted phenomenon, intricately linked to its electronic structure and several other atomic properties. While the number of valence electrons serves as a primary indicator, factors like electronegativity, effective nuclear charge, atomic radius, ionization energy, and electron affinity all play crucial roles in determining an atom's propensity to participate in chemical reactions. A comprehensive understanding of these factors provides a powerful tool for predicting and manipulating chemical behavior, with far-reaching implications across diverse scientific and technological fields. Further research continually refines our understanding of these interactions, leading to advances in materials science, chemical synthesis, environmental science, and biological research. The quest to unravel the complexities of atomic reactivity is an ongoing journey, with discoveries continuously expanding our knowledge and shaping the future of numerous scientific disciplines.
Latest Posts
Latest Posts
-
Which Of The Following Statements About Protein Is Correct
Apr 26, 2025
-
Which Statement Best Describes The Diagram Below
Apr 26, 2025
-
The Sum Of Potential And Kinetic Energy
Apr 26, 2025
-
Which Of The Following Microorganisms Is Prokaryotic
Apr 26, 2025
-
How Many Valence Electrons In Ar
Apr 26, 2025
Related Post
Thank you for visiting our website which covers about The Reactivity Of An Atom Depends On The Number Of . We hope the information provided has been useful to you. Feel free to contact us if you have any questions or need further assistance. See you next time and don't miss to bookmark.