The Physical Expression Of An Organism's Genes
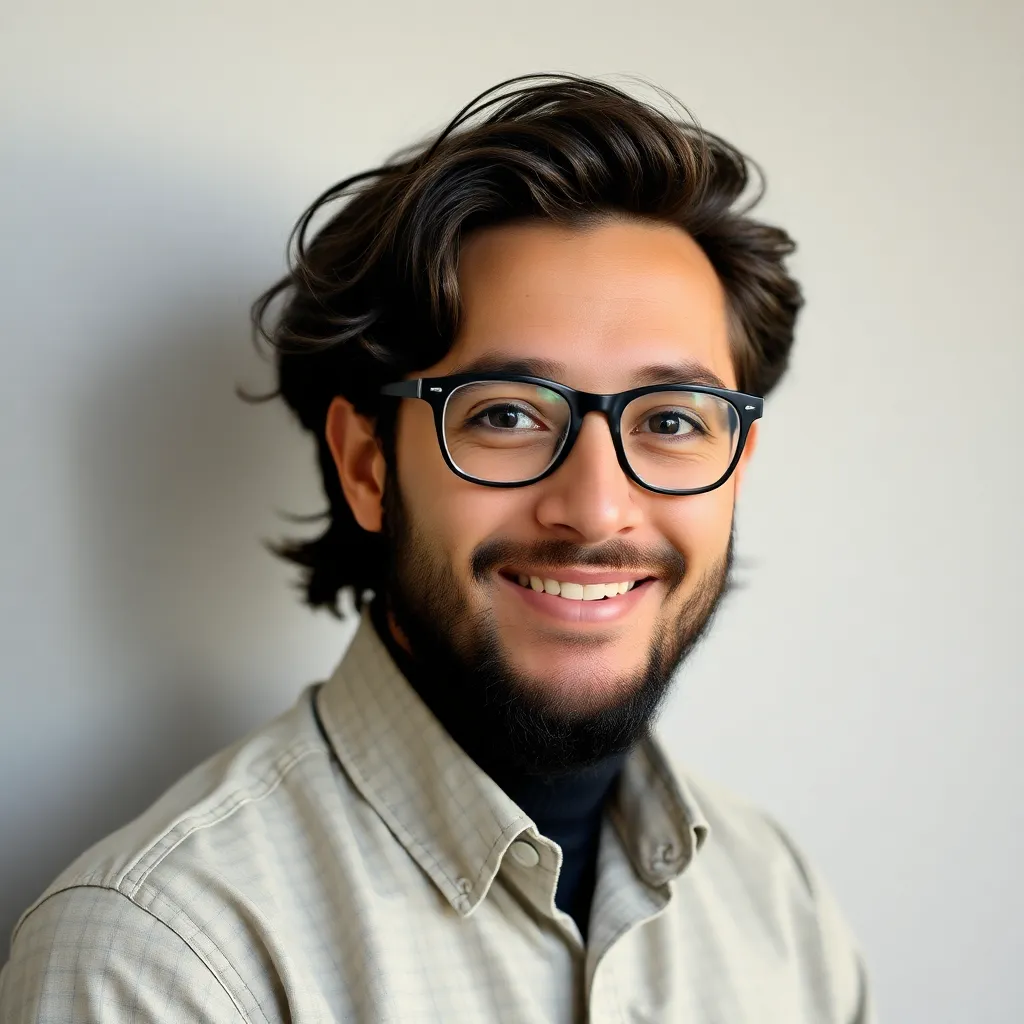
News Leon
Apr 07, 2025 · 7 min read

Table of Contents
The Physical Expression of an Organism's Genes: From Genotype to Phenotype
The intricate dance between an organism's genes and its observable characteristics is a fundamental concept in biology. This relationship, often summarized as the genotype-phenotype connection, is far more complex than a simple one-to-one correspondence. Understanding how genes physically manifest themselves – their expression – is crucial to grasping the diversity of life and the mechanisms underlying inherited traits, diseases, and evolutionary processes. This article delves deep into the multifaceted physical expression of an organism's genes, exploring the processes, influencing factors, and implications of this complex interplay.
The Central Dogma: From DNA to Protein
The central dogma of molecular biology provides the foundational framework for understanding gene expression. It describes the flow of genetic information from DNA (deoxyribonucleic acid), the blueprint of life, to RNA (ribonucleic acid), and finally to proteins, the workhorses of the cell. This process involves two main stages: transcription and translation.
Transcription: DNA to RNA
Transcription is the process where the DNA sequence of a gene is copied into a messenger RNA (mRNA) molecule. This occurs within the cell's nucleus. The enzyme responsible, RNA polymerase, binds to a specific region of the DNA called the promoter, initiating the unwinding of the DNA double helix. RNA polymerase then reads the DNA template strand, synthesizing a complementary mRNA molecule. This mRNA molecule carries the genetic code from the DNA to the ribosomes, the protein synthesis machinery of the cell.
Translation: RNA to Protein
Translation is the process of converting the mRNA sequence into a polypeptide chain, which folds into a functional protein. This takes place in the cytoplasm, primarily at ribosomes. Ribosomes read the mRNA sequence in codons, three-nucleotide units that specify a particular amino acid. Transfer RNA (tRNA) molecules, each carrying a specific amino acid, recognize and bind to the corresponding codons on the mRNA. The ribosome facilitates the formation of peptide bonds between the amino acids, creating a growing polypeptide chain. Once the entire mRNA sequence is translated, the polypeptide chain folds into a specific three-dimensional structure, forming a functional protein.
Beyond the Central Dogma: Epigenetic Modifications
While the central dogma provides a simplified overview, the physical expression of genes is far more nuanced. Epigenetics, the study of heritable changes in gene expression that do not involve alterations to the underlying DNA sequence, plays a significant role. Epigenetic modifications can influence gene expression by altering chromatin structure, the packaging of DNA and proteins within the cell nucleus.
DNA Methylation: Silencing Genes
DNA methylation is a crucial epigenetic modification involving the addition of a methyl group (CH3) to cytosine bases in DNA. This modification often leads to gene silencing, preventing transcription and protein production. DNA methylation patterns can be inherited, influencing gene expression across generations.
Histone Modification: Regulating Accessibility
Histones are proteins around which DNA is wrapped to form chromatin. Histone modification, including acetylation, methylation, and phosphorylation, can alter chromatin structure, influencing the accessibility of DNA to the transcriptional machinery. Histone acetylation, for example, generally loosens chromatin structure, promoting gene expression, while histone methylation can have both activating and repressive effects depending on the specific histone residue modified.
Environmental Influences on Gene Expression
The environment plays a significant role in shaping the physical expression of an organism's genes. Environmental factors such as diet, stress, and exposure to toxins can influence epigenetic modifications and gene regulation. This interplay between genes and the environment underscores the concept of phenotypic plasticity, where an organism's phenotype is influenced by both its genotype and its environment.
Nutritional Influence
Diet can significantly influence gene expression through epigenetic modifications. For instance, certain dietary components can alter DNA methylation patterns, affecting the expression of genes involved in metabolism, immune function, and disease susceptibility.
Stress Response
Stressful experiences can also trigger epigenetic changes, influencing gene expression patterns associated with stress response and mental health. Chronic stress can lead to long-term changes in gene expression, potentially contributing to the development of various diseases.
Exposure to Toxins
Exposure to environmental toxins can also induce epigenetic modifications, altering gene expression and potentially increasing the risk of various health problems. These effects can be long-lasting and even transgenerational, influencing the health of future generations.
Genetic Mutations and their Physical Manifestations
Genetic mutations, changes in the DNA sequence, can have profound effects on gene expression and the resulting phenotype. These mutations can range from single nucleotide polymorphisms (SNPs) to large-scale chromosomal rearrangements.
SNPs and their Effects
SNPs, the most common type of genetic variation, involve changes in a single nucleotide base. While many SNPs have no observable effect, some can alter gene expression by affecting promoter regions, splicing sites, or the coding sequence of a gene. This can lead to changes in protein structure and function, resulting in altered phenotypes.
Chromosomal Abnormalities
Chromosomal abnormalities, such as deletions, duplications, and translocations, can have significant consequences on gene expression. These alterations can disrupt gene regulation, leading to altered phenotypes, often associated with developmental disorders or diseases. Examples include Down syndrome (trisomy 21), Turner syndrome (monosomy X), and various types of cancer.
Gene Regulation Networks and Their Complexity
The physical expression of genes is not a solitary event but rather a coordinated process involving intricate networks of interacting genes and regulatory elements. These networks involve transcription factors, enhancer regions, and other regulatory molecules that influence the timing, location, and level of gene expression.
Transcription Factors: Orchestrating Gene Expression
Transcription factors are proteins that bind to specific DNA sequences, regulating the transcription of target genes. These factors can either activate or repress gene expression, controlling the levels of various proteins within the cell. The intricate interplay of multiple transcription factors orchestrates the precise expression patterns necessary for cellular differentiation, development, and response to environmental stimuli.
Enhancers and Silencers: Fine-Tuning Gene Expression
Enhancers and silencers are DNA sequences that can influence the transcription of genes located at a distance. Enhancers typically activate gene expression, while silencers repress it. These regulatory elements interact with transcription factors and other regulatory molecules to fine-tune gene expression in response to various signals.
The Role of Non-Coding RNAs
Beyond mRNA, various non-coding RNAs (ncRNAs) play crucial roles in regulating gene expression. These ncRNAs do not code for proteins but instead participate in diverse regulatory processes.
MicroRNAs: Fine-Tuning Gene Expression
MicroRNAs (miRNAs) are small ncRNAs that regulate gene expression by binding to complementary sequences in mRNA molecules, leading to mRNA degradation or translational repression. MiRNAs play critical roles in development, differentiation, and disease pathogenesis.
Studying Gene Expression: Techniques and Applications
A range of sophisticated techniques are used to study gene expression, providing insights into the complex interplay between genes and phenotypes. These techniques have widespread applications in various fields, including medicine, agriculture, and biotechnology.
Quantitative PCR (qPCR): Measuring Gene Expression Levels
qPCR is a highly sensitive technique used to quantify the amount of a specific mRNA molecule in a sample. This provides insights into the level of gene expression under different conditions or in different tissues.
Microarray Analysis: Profiling Gene Expression
Microarray technology allows the simultaneous measurement of the expression levels of thousands of genes. This provides a global view of gene expression patterns in a given sample, allowing researchers to identify genes involved in specific biological processes or disease states.
RNA Sequencing (RNA-Seq): Comprehensive Gene Expression Analysis
RNA-Seq is a powerful technique that provides a comprehensive analysis of the transcriptome, the complete set of RNA molecules in a cell or organism. This enables the identification and quantification of all expressed genes, including known and novel transcripts, offering unprecedented detail on gene expression patterns.
Conclusion: The Ever-Evolving Landscape of Gene Expression
The physical expression of an organism's genes is a dynamic and multifaceted process shaped by a complex interplay of factors including the DNA sequence itself, epigenetic modifications, environmental influences, and intricate gene regulatory networks. Understanding this intricate relationship is crucial for advancing our knowledge in various fields. From unraveling the mechanisms underlying human diseases to engineering crops with enhanced yields and developing new therapies, the study of gene expression continues to be a vibrant and rapidly evolving field with far-reaching implications for society. Further research promises to unveil even more intricate details of this remarkable process, offering deeper insights into the complexity and beauty of life itself.
Latest Posts
Latest Posts
-
Which Of The Following Is A Good Conductor Of Heat
Apr 08, 2025
-
Is Gas Evaporating A Chemical Change
Apr 08, 2025
-
Graph Of Kinetic Energy Vs Time
Apr 08, 2025
-
Which Of The Following Is Correctly Paired
Apr 08, 2025
-
Which Of The Following Is True Of Services
Apr 08, 2025
Related Post
Thank you for visiting our website which covers about The Physical Expression Of An Organism's Genes . We hope the information provided has been useful to you. Feel free to contact us if you have any questions or need further assistance. See you next time and don't miss to bookmark.