The Final Electron Acceptor Of The Electron Transport Chain Is
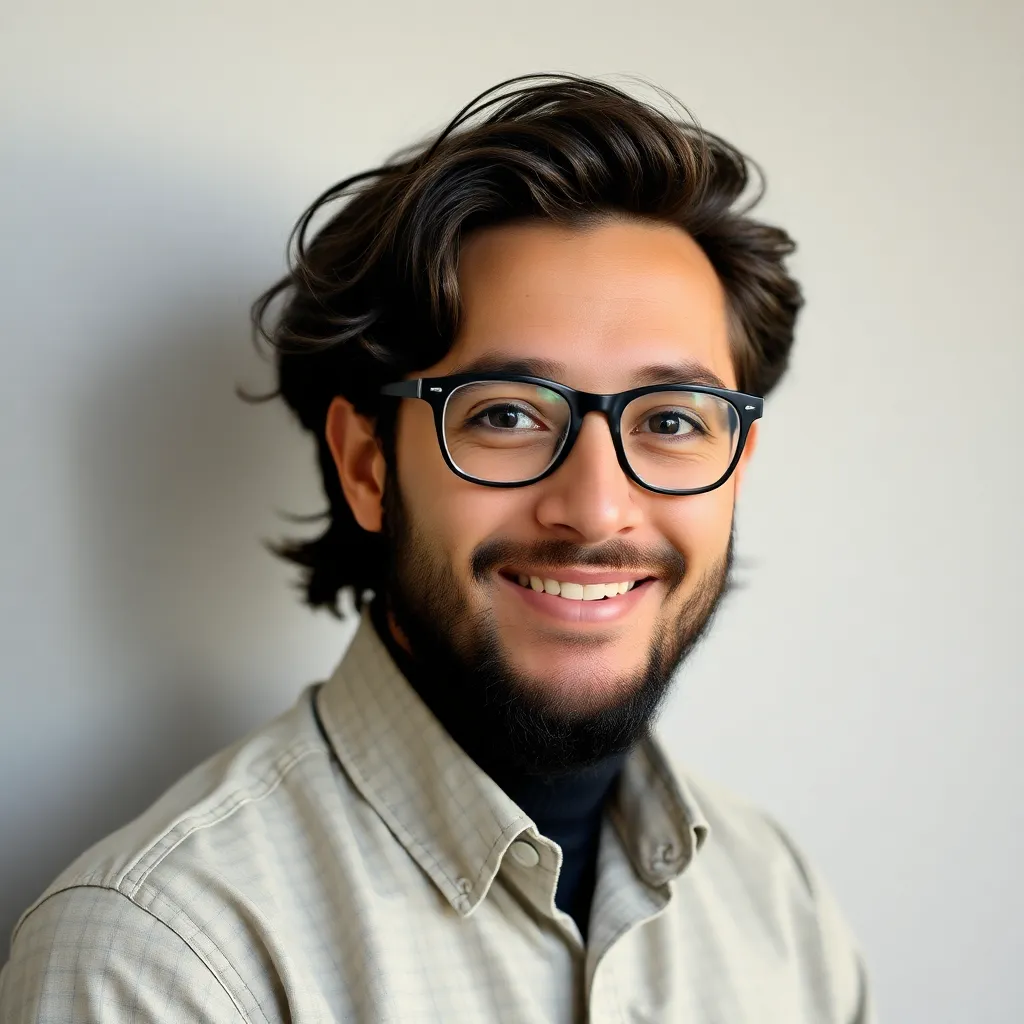
News Leon
Mar 11, 2025 · 7 min read

Table of Contents
The Final Electron Acceptor of the Electron Transport Chain Is… Oxygen! Understanding its Crucial Role in Cellular Respiration
The electron transport chain (ETC), a critical component of cellular respiration, is a series of protein complexes embedded in the inner mitochondrial membrane (in eukaryotes) or the plasma membrane (in prokaryotes). Its primary function is to generate a proton gradient across the membrane, which drives ATP synthesis – the energy currency of the cell. But what makes this intricate process work? The answer lies in the final electron acceptor of the ETC: oxygen (O₂).
Understanding the Electron Transport Chain: A Step-by-Step Breakdown
Before delving into the crucial role of oxygen, let's revisit the fundamental steps of the electron transport chain. The ETC doesn't operate in isolation; it's inextricably linked to the preceding stages of cellular respiration – glycolysis and the Krebs cycle (also known as the citric acid cycle).
These earlier stages break down glucose, extracting high-energy electrons and transferring them to electron carrier molecules, primarily NADH and FADH₂. These carriers then deliver these high-energy electrons to the ETC, initiating the process of oxidative phosphorylation.
The ETC itself is composed of four major protein complexes (Complexes I-IV) and two mobile electron carriers, ubiquinone (CoQ) and cytochrome c. The electrons are passed sequentially through these complexes, moving from a higher energy level to a lower energy level. This controlled electron transfer releases energy, which is harnessed to pump protons (H⁺) across the inner mitochondrial membrane.
Complex I: NADH Dehydrogenase
The journey begins with Complex I, also known as NADH dehydrogenase. Here, NADH donates its high-energy electrons, which are then passed along the chain. This electron transfer is coupled to the pumping of protons from the mitochondrial matrix into the intermembrane space, establishing a proton gradient.
Complex II: Succinate Dehydrogenase
Complex II, succinate dehydrogenase, is slightly different. It's directly involved in the Krebs cycle and receives electrons from FADH₂. Unlike Complex I, Complex II does not pump protons directly. However, it contributes electrons to the ubiquinone pool, which subsequently feeds into the rest of the ETC.
Ubiquinone (CoQ) and Cytochrome c: The Mobile Electron Carriers
Ubiquinone and cytochrome c are small, mobile electron carriers that shuttle electrons between the protein complexes. They facilitate the flow of electrons through the chain, ensuring efficient electron transfer.
Complex III: Cytochrome bc₁ Complex
Complex III, the cytochrome bc₁ complex, receives electrons from ubiquinone and passes them on to cytochrome c. This transfer is also coupled to proton pumping, further increasing the proton gradient across the membrane.
Complex IV: Cytochrome c Oxidase
Finally, the electrons reach Complex IV, cytochrome c oxidase. This complex plays a pivotal role, as it is where oxygen comes in. Oxygen acts as the terminal electron acceptor, meaning it receives the electrons at the end of the chain.
The Indispensable Role of Oxygen as the Final Electron Acceptor
The acceptance of electrons by oxygen is absolutely crucial for the entire process. Without oxygen, the ETC would come to a standstill. This is because the electrons would have nowhere to go, effectively blocking the flow of electrons through the chain and halting proton pumping. This blockage would prevent the formation of the proton gradient, which is essential for ATP synthesis. Therefore, the absence of oxygen leads to a significant reduction in ATP production.
The reaction at Complex IV involves the reduction of oxygen (O₂) to water (H₂O). This is a four-electron reduction, meaning that four electrons are required to reduce one molecule of oxygen to two molecules of water.
O₂ + 4e⁻ + 4H⁺ → 2H₂O
This reaction is highly exergonic, releasing a significant amount of energy that contributes to the overall efficiency of the ETC. The formation of water is also vital, as it prevents the buildup of reactive oxygen species (ROS), which can damage cellular components.
Consequences of Oxygen Depletion: The Shift to Anaerobic Respiration
When oxygen is limited or absent, cells resort to anaerobic respiration (fermentation) to generate ATP. Anaerobic respiration is significantly less efficient than aerobic respiration (which utilizes oxygen), yielding far fewer ATP molecules per glucose molecule. The types of fermentation vary depending on the organism, but they all involve alternative electron acceptors, such as pyruvate or acetaldehyde, instead of oxygen. These alternative pathways are crucial for survival in oxygen-deprived environments but cannot sustain the high energy demands of most organisms over extended periods.
The Significance of Oxygen in Maintaining the Proton Gradient
The electron transfer through the ETC is tightly coupled to proton pumping. As electrons move down the chain, energy is released, and this energy is used to pump protons across the membrane, creating a proton gradient. This gradient is a form of stored energy known as the proton motive force (PMF). The PMF then drives ATP synthesis through the enzyme ATP synthase, located in the inner mitochondrial membrane (or plasma membrane in prokaryotes).
Without the final electron acceptor (oxygen), the electron transport chain would be blocked. This would prevent proton pumping, and the PMF would collapse, dramatically reducing ATP production.
Oxygen's Impact Beyond ATP Production: ROS and Cellular Protection
While oxygen is essential for efficient ATP generation, its involvement also carries a risk. The process of electron transfer in the ETC is not perfectly efficient. Some electrons can leak out and react with oxygen to form reactive oxygen species (ROS). ROS are highly reactive molecules, such as superoxide radicals (O₂⁻) and hydrogen peroxide (H₂O₂), which can damage cellular components, including DNA, proteins, and lipids. This damage is associated with aging and various diseases.
However, cells have evolved sophisticated antioxidant defense mechanisms to mitigate ROS-induced damage. These mechanisms include enzymes such as superoxide dismutase (SOD), catalase, and glutathione peroxidase, which neutralize ROS and protect cellular integrity. The balance between ROS production and antioxidant defense is crucial for maintaining cellular homeostasis.
Exploring Variations in Electron Acceptors Across Different Organisms
While oxygen serves as the terminal electron acceptor in most aerobic organisms, some organisms utilize alternative electron acceptors in anaerobic environments. These organisms are often classified based on their preferred electron acceptor. For example:
- Nitrate-reducing bacteria: utilize nitrate (NO₃⁻) as the terminal electron acceptor, reducing it to nitrite (NO₂⁻) or even nitrogen gas (N₂).
- Sulfate-reducing bacteria: employ sulfate (SO₄²⁻) as the electron acceptor, reducing it to hydrogen sulfide (H₂S).
- Methanogenic archaea: use carbon dioxide (CO₂) to produce methane (CH₄) as a byproduct of their anaerobic metabolism.
These alternative electron acceptors have lower reduction potentials than oxygen, meaning they generate less ATP per electron transferred. This explains why anaerobic respiration is less efficient than aerobic respiration.
The Importance of Oxygen in Evolution and Life on Earth
The evolution of oxygenic photosynthesis, which releases oxygen as a byproduct, fundamentally altered the Earth's atmosphere and paved the way for the development of aerobic respiration. Before the Great Oxidation Event, the Earth's atmosphere was largely anaerobic. The appearance of photosynthetic organisms and the subsequent increase in atmospheric oxygen levels enabled the evolution of more energy-efficient aerobic organisms.
Aerobic respiration, with its reliance on oxygen as the final electron acceptor, is far more efficient than anaerobic respiration. This efficiency provided a selective advantage to organisms capable of using oxygen, leading to their diversification and dominance in many environments.
Conclusion: Oxygen – The Essential Driver of Aerobic Life
In conclusion, oxygen's role as the final electron acceptor in the electron transport chain is absolutely crucial for aerobic life. It allows for the efficient generation of ATP, the primary energy source for cellular processes. Without oxygen, the ETC would be blocked, drastically reducing ATP production and hindering the survival of most organisms. While its utilization comes with the risk of ROS formation, cells have evolved mechanisms to mitigate this danger. Understanding the intricate relationship between the ETC, oxygen, and cellular respiration remains a critical area of research in biology, with implications for medicine, biotechnology, and our understanding of the evolution of life itself.
Latest Posts
Related Post
Thank you for visiting our website which covers about The Final Electron Acceptor Of The Electron Transport Chain Is . We hope the information provided has been useful to you. Feel free to contact us if you have any questions or need further assistance. See you next time and don't miss to bookmark.