The Final Electron Acceptor In The Electron Transport Chain Is:
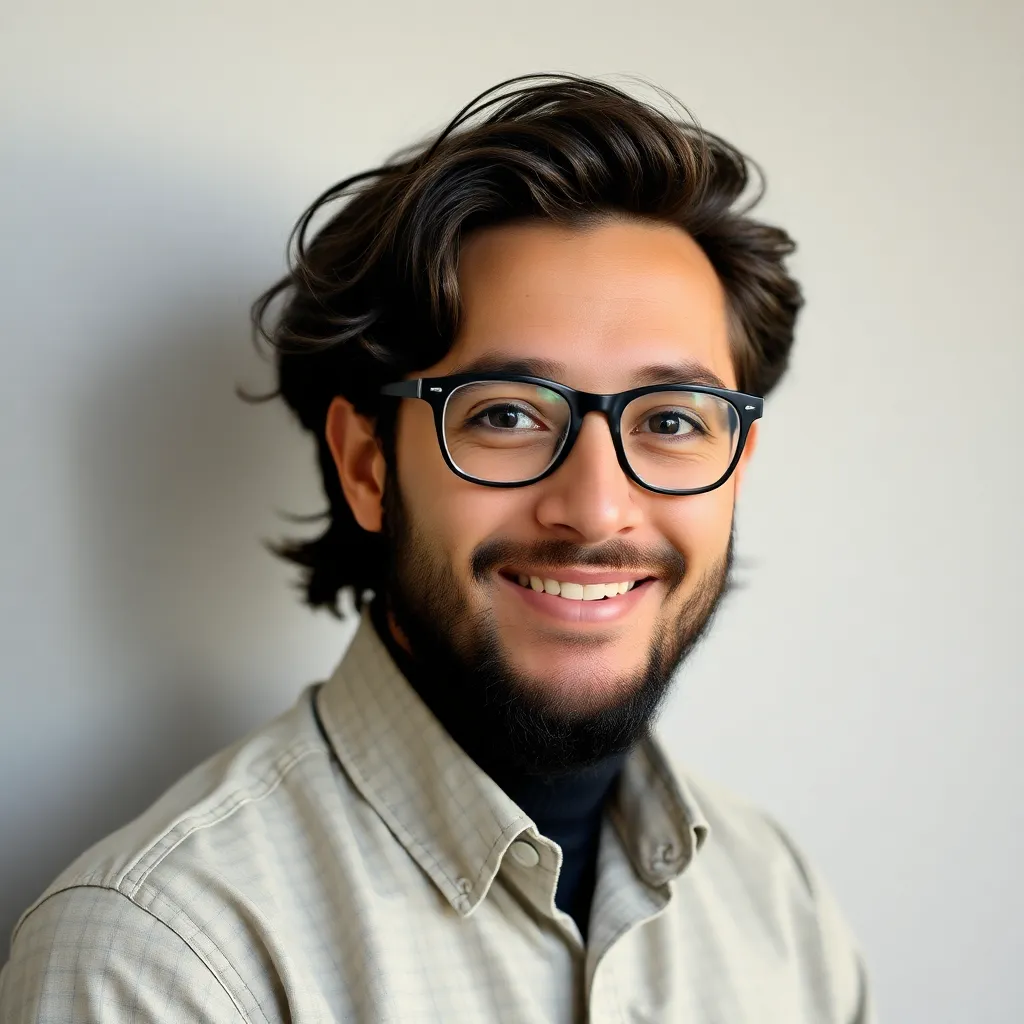
News Leon
Mar 26, 2025 · 6 min read

Table of Contents
The Final Electron Acceptor in the Electron Transport Chain: Oxygen's Crucial Role
The electron transport chain (ETC), also known as the respiratory chain, is a fundamental process in cellular respiration, responsible for generating the majority of the ATP (adenosine triphosphate) that fuels cellular activities. Understanding its intricacies is crucial to comprehending cellular metabolism and energy production. A key player in this intricate process is the final electron acceptor, the molecule that ultimately receives the electrons passed down the chain. This article delves deep into the role of the final electron acceptor, focusing primarily on oxygen, its significance in aerobic respiration, and the consequences of its absence.
Understanding the Electron Transport Chain
The ETC is a series of protein complexes embedded within the inner mitochondrial membrane (in eukaryotes) or the plasma membrane (in prokaryotes). These complexes facilitate the sequential transfer of electrons from electron donors (like NADH and FADH2, generated during glycolysis and the citric acid cycle) to electron acceptors, down a cascade of decreasing redox potential. This electron flow drives the pumping of protons (H+) across the membrane, establishing a proton gradient. This gradient is then harnessed by ATP synthase, an enzyme that uses the flow of protons back across the membrane to synthesize ATP through chemiosmosis.
The Sequential Electron Transfer
The electron transport chain isn't a single, continuous pathway. Instead, it involves four major protein complexes (I-IV in eukaryotes), each with specific roles in electron transport and proton pumping.
- Complex I (NADH dehydrogenase): Receives electrons from NADH, transferring them to ubiquinone (Q), a mobile electron carrier. This transfer is coupled to the pumping of protons.
- Complex II (succinate dehydrogenase): Receives electrons from FADH2, bypassing Complex I and directly donating electrons to ubiquinone. It doesn't pump protons.
- Complex III (cytochrome bc1 complex): Receives electrons from ubiquinone and transfers them to cytochrome c, another mobile electron carrier. This transfer is also coupled to proton pumping.
- Complex IV (cytochrome c oxidase): Receives electrons from cytochrome c and ultimately transfers them to the final electron acceptor. This complex also pumps protons.
This sequential transfer of electrons, coupled with proton pumping, establishes the proton motive force, the driving force behind ATP synthesis.
Oxygen: The Final Electron Acceptor in Aerobic Respiration
In aerobic respiration, the final electron acceptor is molecular oxygen (O2). This is a crucial detail, distinguishing aerobic respiration from anaerobic respiration, which utilizes other molecules as final electron acceptors. The reduction of oxygen to water is the terminal step in the ETC, and this reaction is essential for the continuous flow of electrons.
The Reduction of Oxygen
The reduction of oxygen involves the addition of four electrons, two protons, and ultimately forms water:
1/2 O2 + 2e- + 2H+ → H2O
This reaction is catalyzed by Complex IV (cytochrome c oxidase). The acceptance of electrons by oxygen is crucial because without a final electron acceptor, the ETC would become blocked, halting electron flow and ATP production. The electrons would accumulate in the preceding complexes, preventing further oxidation of NADH and FADH2. This would effectively shut down cellular respiration.
The Importance of Oxygen in ATP Production
The role of oxygen as the final electron acceptor is inextricably linked to the high efficiency of aerobic respiration. Oxygen's high electronegativity makes it an excellent electron acceptor, allowing for a large drop in free energy during electron transport. This substantial free energy change is what drives the efficient production of ATP. Without oxygen, the yield of ATP would be significantly lower.
Comparison with Anaerobic Respiration
Anaerobic respiration uses alternative electron acceptors, such as sulfate (SO42-), nitrate (NO3-), or fumarate. These acceptors have lower redox potentials than oxygen, meaning the free energy change during electron transport is smaller. Consequently, anaerobic respiration generates significantly less ATP than aerobic respiration.
This reduced ATP production is one of the reasons why aerobic organisms thrive in oxygen-rich environments. The high energy yield of aerobic respiration provides them with a significant metabolic advantage over organisms reliant on anaerobic respiration.
Consequences of Oxygen Absence: The Shift to Anaerobic Metabolism
When oxygen is unavailable, cells must shift to anaerobic metabolic pathways to maintain energy production. This shift typically involves fermentation, a process that doesn't involve the ETC but regenerates NAD+ from NADH, allowing glycolysis to continue. However, fermentation produces far less ATP than aerobic respiration.
Fermentation: A Less Efficient Alternative
Fermentation processes, like lactic acid fermentation in muscle cells or alcoholic fermentation in yeast, produce only two ATP molecules per glucose molecule, compared to the approximately 30-36 ATP molecules generated during aerobic respiration. This significant difference in ATP yield highlights the importance of oxygen in efficient energy production.
The Build-up of Metabolic Byproducts
Another consequence of oxygen absence is the accumulation of metabolic byproducts. In lactic acid fermentation, lactic acid accumulates, causing muscle fatigue and soreness. In alcoholic fermentation, ethanol and carbon dioxide are produced. These byproducts can have negative impacts on cellular function if they accumulate to high levels. The body's ability to handle these byproducts is limited, and their accumulation contributes to the overall limitations of anaerobic metabolism.
Oxygen Toxicity: The Other Side of the Coin
While oxygen is essential for aerobic respiration, it's also a reactive molecule that can generate harmful reactive oxygen species (ROS), such as superoxide radicals (O2-) and hydrogen peroxide (H2O2). These ROS can damage cellular components, including proteins, lipids, and DNA, leading to oxidative stress and potentially contributing to aging and various diseases.
Cellular Defenses Against ROS
Cells have evolved sophisticated mechanisms to counteract ROS, including antioxidant enzymes like superoxide dismutase (SOD) and catalase. These enzymes convert ROS into less harmful molecules, protecting cells from oxidative damage. However, the balance between ROS production and antioxidant defenses is crucial; an imbalance can lead to significant cellular damage.
The Final Electron Acceptor and Evolutionary Significance
The evolution of the ability to use oxygen as the final electron acceptor was a pivotal event in the history of life on Earth. This transition from anaerobic to aerobic respiration significantly increased the efficiency of energy production, allowing for the evolution of larger, more complex organisms. The increased energy availability provided by aerobic respiration fueled the diversification of life and the development of multicellular organisms.
The Great Oxidation Event
The "Great Oxidation Event," approximately 2.4 billion years ago, marked a dramatic shift in Earth's atmosphere, with the accumulation of oxygen produced by photosynthetic cyanobacteria. This increase in atmospheric oxygen dramatically changed the selective pressures on life, favoring organisms that could utilize oxygen for respiration. This transition had profound implications for the evolution of life and the development of the biosphere as we know it.
Conclusion: Oxygen's Irreplaceable Role
In conclusion, oxygen serves as the crucial final electron acceptor in the electron transport chain of aerobic respiration. Its high electronegativity allows for efficient electron transfer and maximal ATP production. The absence of oxygen forces a switch to anaerobic metabolism, resulting in significantly reduced ATP production and the accumulation of metabolic byproducts. Understanding the importance of oxygen as the final electron acceptor is fundamental to understanding cellular respiration, energy production, and the evolution of life itself. The intricate balance between oxygen's essential role and its potential for generating harmful ROS further highlights its complex and vital role in biological systems. Further research continues to unravel the complexities of the ETC and its interaction with oxygen, offering insights into various physiological processes and disease mechanisms.
Latest Posts
Related Post
Thank you for visiting our website which covers about The Final Electron Acceptor In The Electron Transport Chain Is: . We hope the information provided has been useful to you. Feel free to contact us if you have any questions or need further assistance. See you next time and don't miss to bookmark.