The Chemical Reactivity Of An Element Is Dependent On
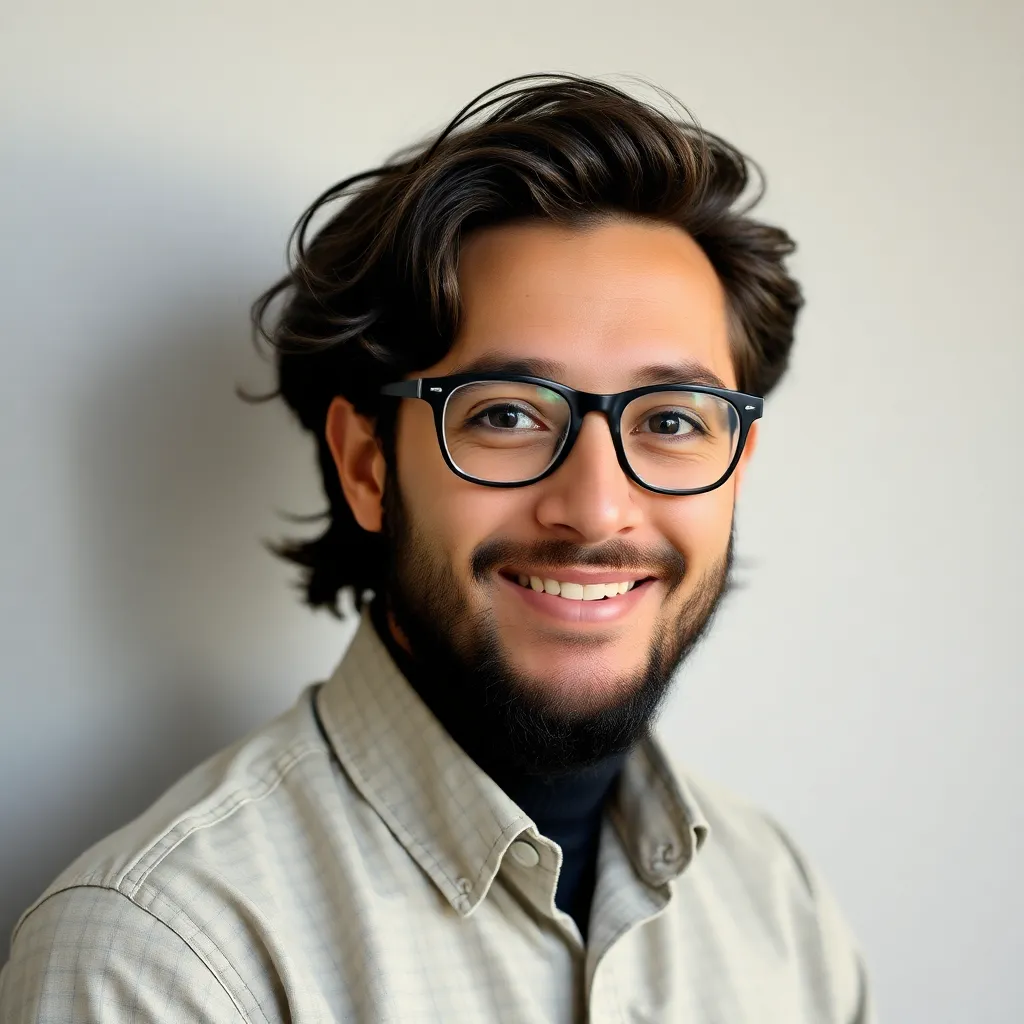
News Leon
Apr 11, 2025 · 6 min read

Table of Contents
The Chemical Reactivity of an Element is Dependent On: A Deep Dive into Atomic Structure and Bonding
The chemical reactivity of an element, its propensity to undergo chemical changes and form new substances, is not a random occurrence. It's a meticulously orchestrated dance dictated by the intricate arrangement of subatomic particles within its atoms. Understanding an element's reactivity requires delving into the fundamentals of atomic structure, specifically its electron configuration and the resulting tendency to gain, lose, or share electrons to achieve stability. This article explores the key factors influencing an element's chemical behavior, providing a comprehensive overview of the underlying principles.
1. Electron Configuration: The Foundation of Reactivity
At the heart of an element's reactivity lies its electron configuration, the arrangement of electrons in its various energy levels and sublevels. Electrons occupy orbitals, regions of space around the nucleus where there's a high probability of finding them. These orbitals are organized into shells (principal energy levels) and subshells (s, p, d, and f orbitals). The outermost shell, containing the valence electrons, plays the most crucial role in determining reactivity.
1.1 Valence Electrons: The Key Players
Valence electrons are the electrons in the outermost shell of an atom. They are the electrons most likely to participate in chemical bonding because they experience the least attraction from the nucleus. Atoms strive to achieve a stable electron configuration, often resembling that of a noble gas (Group 18 elements). This stable configuration typically involves a full outermost shell, often eight electrons (the octet rule, with some exceptions).
1.2 Octet Rule and Exceptions: Understanding Stability
The octet rule states that atoms tend to gain, lose, or share electrons to achieve a full valence shell of eight electrons. This stable configuration minimizes their potential energy, making them less reactive. However, there are exceptions to the octet rule, particularly for elements in the third period and beyond, where expanded valence shells are possible. For example, phosphorus can form compounds with more than eight valence electrons.
1.3 Electron Affinity and Ionization Energy: Quantifying Reactivity
Two crucial properties quantify an atom's tendency to gain or lose electrons:
- Electron Affinity: This measures the energy change when an atom gains an electron. A high electron affinity indicates a strong tendency to accept electrons, characteristic of nonmetals.
- Ionization Energy: This measures the energy required to remove an electron from an atom. A low ionization energy signifies a strong tendency to lose electrons, typical of metals.
2. Electronegativity: Sharing the Electrons
Electronegativity is a measure of an atom's ability to attract electrons in a chemical bond. It's a crucial factor influencing the type of bond formed between atoms. Elements with high electronegativity strongly attract electrons, while elements with low electronegativity have a weaker pull on electrons.
2.1 Types of Chemical Bonds: Ionic, Covalent, and Metallic
The difference in electronegativity between two atoms dictates the type of bond they form:
-
Ionic Bonds: Formed when there's a large electronegativity difference, resulting in the transfer of electrons from one atom to another. This creates ions—positively charged cations (metal) and negatively charged anions (nonmetal)—held together by electrostatic attraction. Ionic compounds are typically formed between metals and nonmetals.
-
Covalent Bonds: Formed when atoms share electrons to achieve a stable electron configuration. Covalent bonds occur between nonmetals, where the electronegativity difference is small. The shared electrons are attracted to the nuclei of both atoms. The degree of sharing can vary, leading to polar and nonpolar covalent bonds.
-
Metallic Bonds: Found in metals, where valence electrons are delocalized and move freely throughout the metal lattice. This creates a "sea" of electrons that holds the positively charged metal ions together. This is why metals are good conductors of electricity and heat.
3. Atomic Size and Shielding Effect: Influence on Reactivity
The atomic size and the shielding effect significantly influence an element's reactivity.
3.1 Atomic Radius: Distance Matters
Atomic radius refers to the size of an atom. As you move down a group in the periodic table, atomic size increases due to the addition of electron shells. Larger atoms have valence electrons farther from the nucleus, experiencing weaker attraction. This makes them more readily available for bonding, increasing reactivity. Conversely, moving across a period, atomic size generally decreases, leading to increased attraction and decreased reactivity.
3.2 Shielding Effect: Inner Electrons' Influence
The shielding effect refers to the reduction in the attraction between the nucleus and valence electrons caused by inner electrons. Inner electrons repel valence electrons, reducing the effective nuclear charge experienced by valence electrons. This effect becomes more significant as you move down a group, leading to an increase in atomic size and reactivity.
4. Periodic Trends and Reactivity: Organizing the Elements
The periodic table beautifully organizes elements based on their properties, including reactivity. Understanding periodic trends helps predict an element's chemical behavior.
4.1 Group Trends: Similar Reactivities
Elements within the same group (vertical column) have similar electron configurations and valence electron numbers, resulting in similar chemical properties and reactivity. For instance:
-
Group 1 (Alkali Metals): Highly reactive due to their single valence electron readily lost to form +1 ions. Reactivity increases down the group due to increasing atomic size.
-
Group 2 (Alkaline Earth Metals): Reactive, but less so than alkali metals, due to their two valence electrons. Reactivity increases down the group.
-
Group 17 (Halogens): Highly reactive nonmetals, readily gaining one electron to form -1 ions. Reactivity decreases down the group.
-
Group 18 (Noble Gases): Inert (unreactive) due to their complete valence shells.
4.2 Period Trends: Varying Reactivities
Moving across a period (horizontal row), reactivity trends vary. Metals on the left side tend to be more reactive, while nonmetals on the right side are also reactive but in a different manner (gaining electrons). The noble gases at the far right are generally inert.
5. Oxidation States and Reactivity: Charge and Bonding
An element's oxidation state (or oxidation number) represents the hypothetical charge an atom would have if all bonds were completely ionic. It's a useful concept to understand reactivity and predict the products of chemical reactions. Elements with multiple possible oxidation states often exhibit variable reactivity, capable of forming various compounds.
6. Factors Affecting Reaction Rates: Beyond Intrinsic Properties
While an element's intrinsic properties (electron configuration, electronegativity, etc.) dictate its potential for reactivity, the actual rate of a reaction is influenced by several other factors:
-
Concentration: Higher concentrations of reactants lead to more frequent collisions and faster reactions.
-
Temperature: Increased temperature provides reactants with more kinetic energy, increasing the frequency and energy of collisions, leading to faster reactions.
-
Surface Area: For reactions involving solids, a larger surface area exposes more reactant particles, increasing the rate of reaction.
-
Presence of a Catalyst: Catalysts increase reaction rates by providing an alternative reaction pathway with lower activation energy.
7. Conclusion: A Complex Interplay
The chemical reactivity of an element is a multifaceted phenomenon, a complex interplay of atomic structure, bonding characteristics, and reaction conditions. Understanding the fundamental principles governing electron configuration, electronegativity, atomic size, and periodic trends provides a solid foundation for predicting and interpreting chemical behavior. While intrinsic properties determine an element's potential for reactivity, external factors significantly influence the actual rate at which reactions occur. This intricate relationship between atomic structure and chemical behavior is a cornerstone of chemistry, providing a framework for understanding the vast diversity of chemical reactions and the properties of matter.
Latest Posts
Latest Posts
-
How Many Bonds Are Between Adenine And Thymine
Apr 18, 2025
-
Are Seismic Waves Mechanical Or Electromagnetic
Apr 18, 2025
-
Equal Volumes Of 0 2 M Solutions Of Lead
Apr 18, 2025
-
Which Process Is Absent In Animal Cells
Apr 18, 2025
-
What Is The Value Of Y In This Triangle
Apr 18, 2025
Related Post
Thank you for visiting our website which covers about The Chemical Reactivity Of An Element Is Dependent On . We hope the information provided has been useful to you. Feel free to contact us if you have any questions or need further assistance. See you next time and don't miss to bookmark.