Secondary Structure Of Proteins Are Stabilized By
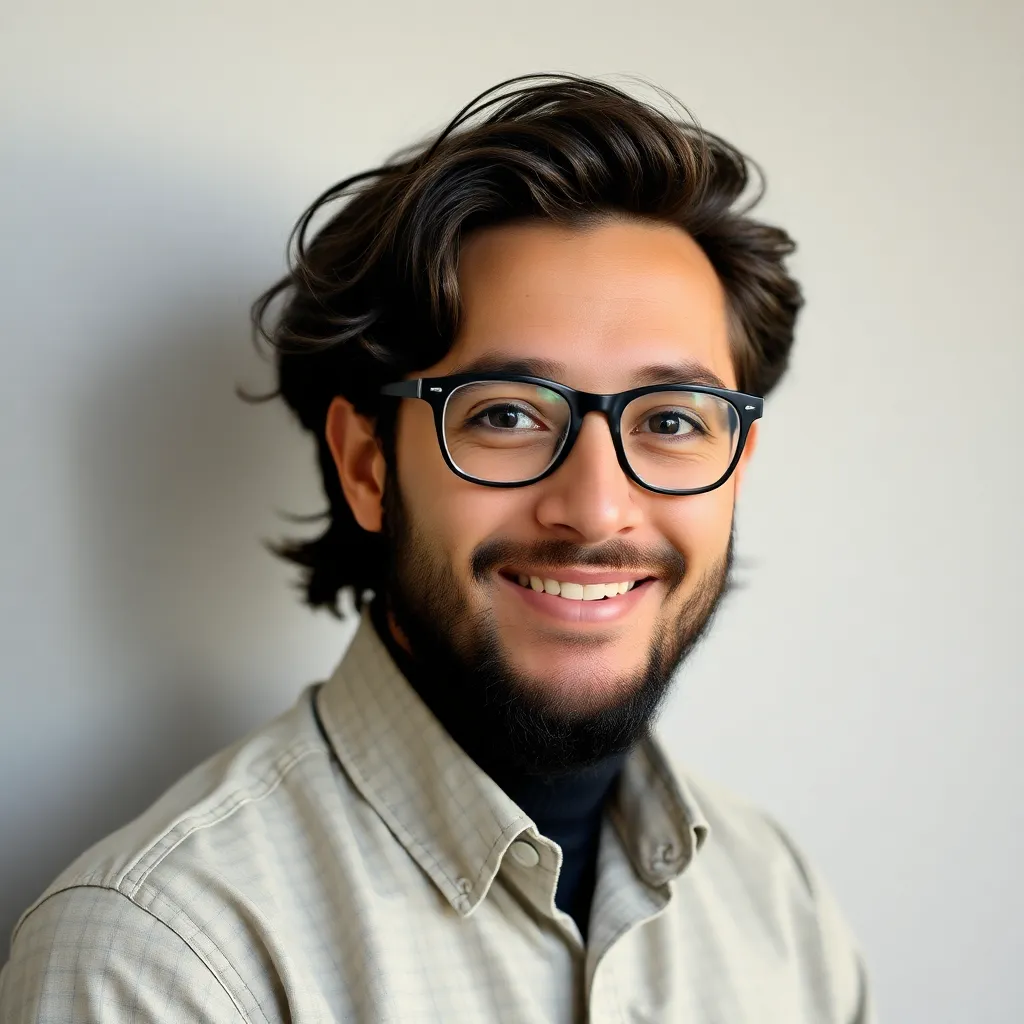
News Leon
Mar 09, 2025 · 7 min read

Table of Contents
Secondary Structure of Proteins: Stabilized by Hydrogen Bonds and More
Proteins, the workhorses of the cell, perform a vast array of functions, from catalyzing biochemical reactions to providing structural support. Their incredible versatility stems from their unique three-dimensional structures, which are ultimately determined by their amino acid sequences. This intricate architecture is built hierarchically, starting with the secondary structure, which forms the foundation for the more complex tertiary and quaternary structures. Understanding how secondary structures are stabilized is crucial to comprehending protein function and the consequences of mutations or environmental changes.
The Building Blocks: Understanding Secondary Structures
Before delving into the forces that stabilize secondary structures, let's briefly revisit what they are. Secondary structures refer to the local, regular folding patterns within a polypeptide chain. These patterns arise from hydrogen bonding between the backbone amide and carbonyl groups of amino acids. The two most common secondary structures are:
1. Alpha-Helices
Alpha-helices are right-handed coiled structures, resembling a spiral staircase. The carbonyl oxygen of each amino acid forms a hydrogen bond with the amide hydrogen of the amino acid four residues down the chain. This regular pattern of hydrogen bonds creates a stable, rod-like structure. The R-groups of the amino acids extend outward from the helix, influencing its properties and interactions with other molecules. The stability of an alpha-helix is significantly impacted by the amino acid sequence. Certain amino acids, like proline (due to its rigid ring structure) and glycine (due to its high flexibility), are helix breakers, while others, such as alanine and leucine, are helix formers.
2. Beta-Sheets
Beta-sheets are formed by hydrogen bonding between extended polypeptide chains, arranged either parallel or antiparallel to each other. In a parallel beta-sheet, the strands run in the same direction (N-terminus to C-terminus), resulting in bent hydrogen bonds. In an antiparallel beta-sheet, the strands run in opposite directions, resulting in straighter, stronger hydrogen bonds. Like alpha-helices, the R-groups of the amino acids project above and below the plane of the sheet. The size and shape of these R-groups can influence the overall stability of the beta-sheet. Similarly, certain amino acids show a preference for beta-sheet formation, while others disfavor it.
The Primary Stabilizing Force: Hydrogen Bonds
The most significant force responsible for stabilizing both alpha-helices and beta-sheets is hydrogen bonding. These relatively weak bonds, formed between the partially positive hydrogen atom of an amide group and the partially negative oxygen atom of a carbonyl group, collectively contribute considerable stability to the secondary structure. The precise geometry of these hydrogen bonds is crucial for optimal stability; deviations from ideal bond angles and distances can weaken the structure. The abundance of hydrogen bonds in these secondary structures is a key factor contributing to their relative stability compared to a completely unfolded polypeptide chain.
Other Contributing Factors to Secondary Structure Stability
While hydrogen bonds are paramount, other forces also play crucial roles in stabilizing secondary structures:
1. Steric Effects and Van der Waals Interactions
The spatial arrangement of amino acid side chains (R-groups) influences the stability of secondary structures. Steric clashes, where bulky R-groups are forced too close together, can destabilize the structure. Conversely, favorable van der Waals interactions between closely packed R-groups can contribute to stability. These weak, short-range forces, arising from temporary fluctuations in electron distribution, cumulatively contribute to the overall energy landscape of the protein. Optimizing these interactions is essential for achieving a stable secondary structure.
2. Peptide Bond Planarity
The peptide bond, connecting adjacent amino acids, exhibits partial double-bond character due to resonance. This restricts rotation around the peptide bond, essentially keeping the six atoms of the peptide group (Cα-CO-NH-Cα) planar. This planarity is a crucial constraint that influences the possible conformations a polypeptide chain can adopt, favoring the formation of alpha-helices and beta-sheets.
3. The Role of the Solvent: Hydrophobic Interactions
The surrounding environment, particularly the solvent, significantly influences protein folding and stability. In an aqueous environment, hydrophobic amino acid side chains tend to cluster together in the protein's interior, minimizing their contact with water. This phenomenon, known as the hydrophobic effect, contributes to the overall stability of the folded protein, indirectly influencing the stability of secondary structures by dictating the overall arrangement of amino acids. This effect plays a role in directing hydrophobic residues towards the core of alpha-helices and beta-sheets, further enhancing stability.
4. Electrostatic Interactions
Charged amino acid side chains can interact with each other through electrostatic interactions (ionic bonds or salt bridges). These interactions, whether attractive (between oppositely charged residues) or repulsive (between similarly charged residues), can significantly influence the stability of the secondary structure. Attractive electrostatic interactions can stabilize the structure, while repulsive interactions can destabilize it. The positioning of charged residues within the secondary structure is crucial for maintaining stability. The dielectric constant of the surrounding medium also modulates the strength of these interactions.
The Impact of Amino Acid Sequence on Stability
The amino acid sequence dictates the propensity of a polypeptide chain to form specific secondary structures. Certain amino acids, as mentioned earlier, are particularly favorable or unfavorable for alpha-helix or beta-sheet formation. For example, alanine and leucine are strong helix formers, while proline and glycine are helix breakers. The sequence also determines the occurrence of other less common secondary structures, such as 3<sub>10</sub> helices and π-helices, which are variations on the alpha-helix theme. The distribution of charged, polar, and hydrophobic amino acids along the sequence influences the folding pathway and the final stability of the protein, impacting the conformation and stability of its secondary structure elements.
Disrupting Secondary Structure: Denaturation
Secondary structures are not immutable; they can be disrupted by various factors, a process known as denaturation. Factors that can lead to denaturation include:
- High temperature: Increased thermal energy disrupts the weak hydrogen bonds and other interactions that stabilize secondary structures.
- Changes in pH: Extreme pH values can alter the charge distribution on amino acid side chains, disrupting electrostatic interactions and potentially destabilizing the structure.
- Organic solvents: Organic solvents can interfere with hydrogen bonding and hydrophobic interactions, leading to denaturation.
- Chaotropic agents: These agents, such as urea and guanidinium chloride, disrupt the hydrogen bonding network in water, thereby reducing the stability of the protein's secondary structures.
The Importance of Secondary Structure in Protein Function
The secondary structures of proteins aren't merely structural elements; they are critical determinants of protein function. The specific arrangement of alpha-helices and beta-sheets creates a unique three-dimensional scaffold that determines the protein's overall shape and its ability to interact with other molecules. Alpha-helices are often found in transmembrane proteins, where they span the lipid bilayer, while beta-sheets are frequently involved in protein-protein interactions. The precise arrangement of secondary structure elements dictates the location of active sites in enzymes, binding sites for ligands, and interaction surfaces for other proteins. Any disruption to secondary structure, such as through denaturation or mutation, can significantly impair or completely abolish protein function.
Conclusion
The stability of secondary structures in proteins is a multifaceted phenomenon governed by a complex interplay of forces. While hydrogen bonds are the primary stabilizing force, steric effects, van der Waals interactions, the hydrophobic effect, electrostatic interactions, and the planarity of the peptide bond all contribute significantly to the overall stability. Understanding these interactions is critical for comprehending protein folding, function, and the impact of mutations and environmental changes. The remarkable stability of secondary structures, resulting from the intricate balance of these forces, underlies the diverse and essential roles proteins play in living organisms. The intricate dance of these forces ensures the precise formation and maintenance of the protein's functional architecture, making it a fascinating area of ongoing research in structural biology and biochemistry. Further research continues to unravel the finer details of these interactions, leading to a deeper appreciation of the remarkable complexity and elegance of protein structure and function.
Latest Posts
Related Post
Thank you for visiting our website which covers about Secondary Structure Of Proteins Are Stabilized By . We hope the information provided has been useful to you. Feel free to contact us if you have any questions or need further assistance. See you next time and don't miss to bookmark.