Rungs Of The Dna Ladder Made Of
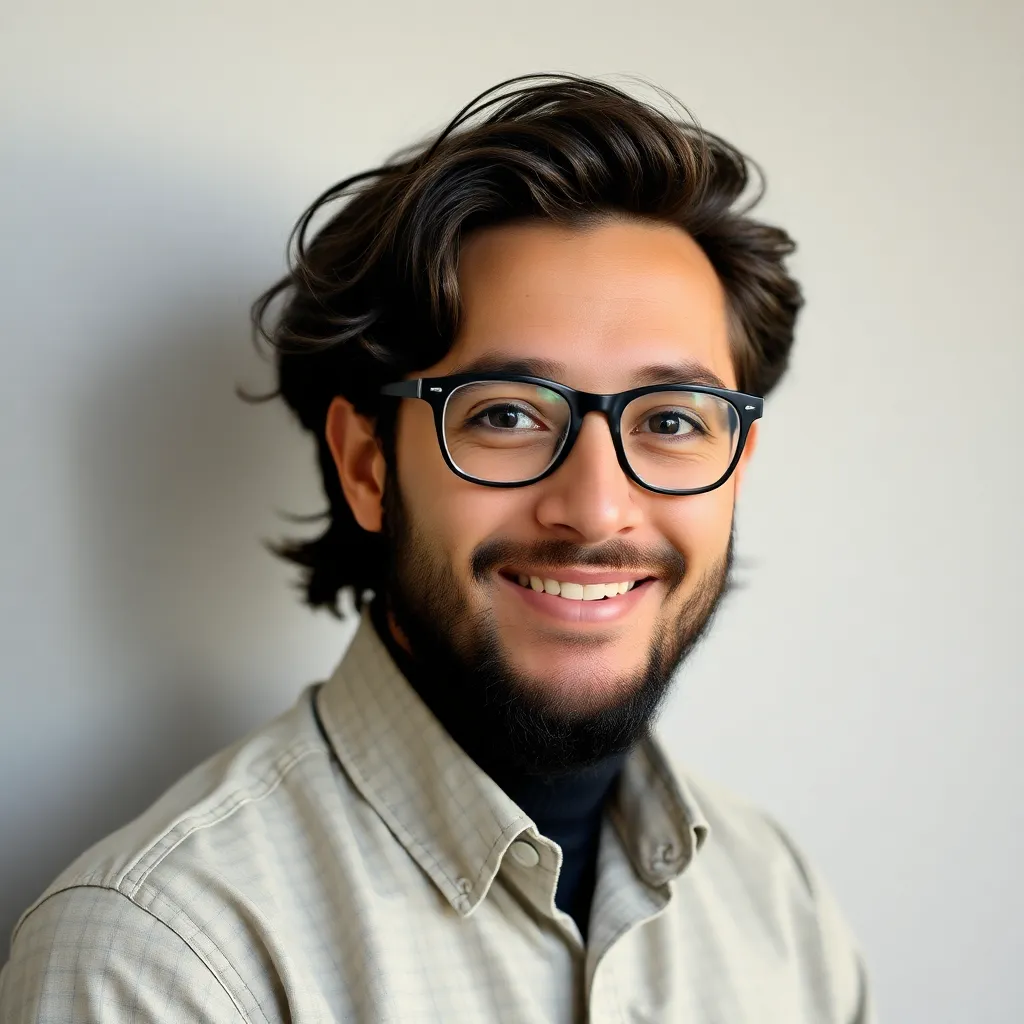
News Leon
Mar 13, 2025 · 7 min read

Table of Contents
The Rungs of the DNA Ladder: A Deep Dive into Nucleotide Base Pairs
The iconic double helix structure of DNA, often depicted as a twisted ladder, is a marvel of nature. While the backbone of this ladder, formed by sugar and phosphate molecules, provides structural support, it's the rungs that hold the genetic code itself – the blueprint for life. These rungs are formed by pairs of nucleotides, specifically the nitrogenous bases: adenine (A), thymine (T), guanine (G), and cytosine (C). The precise pairing of these bases is crucial for DNA replication, transcription, and ultimately, the expression of genetic information. This article will delve into the intricate details of these base pairs, exploring their chemical structures, bonding mechanisms, and significance in biological processes.
The Four Nitrogenous Bases: A Closer Look
Before examining the pairings, let's individually analyze the four key players:
-
Adenine (A): Adenine is a purine base, meaning it contains a double-ring structure. This structure is composed of a six-membered ring fused to a five-membered ring, both containing nitrogen atoms. The specific arrangement of atoms and bonds within this structure allows adenine to form specific interactions with its complementary base.
-
Thymine (T): Thymine is a pyrimidine base, possessing a single six-membered ring structure containing nitrogen atoms. Its structure is distinctly different from adenine's, which is critical for its selective pairing.
-
Guanine (G): Guanine, like adenine, is a purine base with a double-ring structure. However, its arrangement of atoms and functional groups differs from adenine's, leading to its unique pairing properties.
-
Cytosine (C): Cytosine, similar to thymine, is a pyrimidine base with a single six-membered ring structure. Again, the specific arrangement of atoms and functional groups distinguishes it from thymine and determines its pairing specificity.
The Magic of Base Pairing: Hydrogen Bonds and Molecular Specificity
The rungs of the DNA ladder are formed by the precise pairing of these bases through hydrogen bonds. Hydrogen bonds are relatively weak bonds compared to covalent bonds, but their collective strength in the vast network of DNA base pairs contributes significantly to the stability of the double helix. Crucially, the specificity of base pairing arises from the precise geometry and arrangement of hydrogen bond donor and acceptor sites on each base.
-
Adenine (A) always pairs with Thymine (T): A and T form two hydrogen bonds between them. The specific positions of hydrogen atoms and electronegative atoms (oxygen and nitrogen) allow for the optimal formation of these two hydrogen bonds. Any other pairing would be sterically hindered and energetically unfavorable.
-
Guanine (G) always pairs with Cytosine (C): G and C form three hydrogen bonds. The greater number of hydrogen bonds between G and C contributes to the stronger stability of G-C base pairs compared to A-T base pairs. This difference in bond strength has implications for the melting temperature of DNA, which is the temperature at which the double helix separates into single strands.
The complementarity of base pairing is fundamental to DNA's function. The sequence of bases on one strand dictates the sequence on the complementary strand. This principle is essential for DNA replication, where each strand serves as a template for the synthesis of a new, complementary strand.
Beyond the Basic Pairing: Variations and Implications
While A-T and G-C are the standard base pairs in DNA, variations exist, particularly in RNA. RNA, which plays a crucial role in protein synthesis, uses uracil (U) instead of thymine. Uracil, a pyrimidine base, pairs with adenine (A) through two hydrogen bonds, similar to the A-T pairing in DNA. This substitution has functional implications for RNA's roles in transcription and translation.
Beyond the standard pairings, other interactions can occur between bases, though they are less common and typically involve non-canonical pairings. These non-canonical pairings can play roles in specific DNA structures like hairpin loops or in interactions between DNA and proteins.
The Significance of Base Pairing in Biological Processes
The precise and complementary nature of DNA base pairing is crucial for several essential biological processes:
-
DNA Replication: During DNA replication, the double helix unwinds, and each strand serves as a template for the synthesis of a new complementary strand. The fidelity of replication hinges on the accurate pairing of bases, ensuring that genetic information is passed on accurately to daughter cells. Errors in base pairing can lead to mutations, which can have significant consequences for the organism.
-
Transcription: Transcription is the process of creating an RNA copy of a DNA sequence. RNA polymerase, an enzyme, binds to DNA and synthesizes a complementary RNA strand using the DNA strand as a template. Accurate base pairing ensures the faithful transcription of genetic information into RNA molecules.
-
Translation: Translation is the process of synthesizing proteins from the information encoded in mRNA (messenger RNA). During translation, the sequence of codons (three-base sequences) in mRNA is translated into a sequence of amino acids, which form the building blocks of proteins. The accuracy of this process relies on the recognition of codons by their corresponding anticodons in tRNA (transfer RNA) molecules. This recognition also involves base pairing, ensuring the correct amino acids are incorporated into the growing protein chain.
-
DNA Repair Mechanisms: The cell possesses sophisticated repair mechanisms to correct errors that occur during DNA replication or due to environmental factors. Many of these mechanisms rely on recognizing and correcting mismatched base pairs. The cell's ability to detect and correct these errors is essential for maintaining genome stability.
Base Pairing and Disease: Mutations and their Consequences
Errors in base pairing can lead to mutations, which are alterations in the DNA sequence. Mutations can arise spontaneously or be induced by external factors such as radiation or certain chemicals. These mutations can have various consequences:
-
Silent Mutations: Some mutations do not change the amino acid sequence of a protein, owing to the redundancy of the genetic code. These silent mutations generally have no phenotypic effect.
-
Missense Mutations: Other mutations alter a single amino acid in a protein. The effect of a missense mutation depends on the specific amino acid change and its location within the protein. Some missense mutations may have subtle effects, while others can drastically alter protein function.
-
Nonsense Mutations: Nonsense mutations create premature stop codons, leading to truncated, non-functional proteins. These mutations often have severe consequences.
-
Frameshift Mutations: Frameshift mutations occur when the reading frame of the DNA sequence is shifted due to insertions or deletions of nucleotides. These mutations drastically alter the amino acid sequence downstream of the mutation, typically resulting in non-functional proteins.
The impact of mutations varies greatly depending on the specific gene affected and the nature of the mutation. Many genetic diseases are caused by mutations in specific genes, highlighting the critical importance of accurate base pairing in maintaining genome integrity and health.
Advanced Concepts and Future Directions
The field of DNA research continues to evolve, uncovering new layers of complexity in base pairing and its implications. Some advanced concepts and areas of ongoing research include:
-
Non-canonical Base Pairs: The exploration of non-canonical base pairs and their roles in DNA structure and function is an active area of research. These unusual pairings may play roles in gene regulation and other biological processes.
-
DNA Nanotechnology: Researchers are exploring the use of DNA's precise base pairing properties in nanotechnology applications, including the construction of nanoscale structures and devices.
-
Epigenetics: Epigenetic modifications, such as DNA methylation, can alter gene expression without changing the underlying DNA sequence. These modifications can influence base pairing interactions and have significant implications for development and disease.
-
Base Editing: Base editing technologies allow researchers to precisely modify specific bases in the genome without creating double-strand breaks. These techniques hold promise for treating genetic diseases by correcting disease-causing mutations.
Conclusion: The Foundation of Life
The rungs of the DNA ladder, formed by the precise pairing of adenine with thymine and guanine with cytosine, are the very foundation of life. Their intricate structure and specific interactions underpin the fundamental processes of DNA replication, transcription, and translation, ensuring the accurate transmission and expression of genetic information. A deep understanding of base pairing is not only essential for comprehending the intricacies of molecular biology but also for advancing medical technologies and developing new therapies for genetic diseases. The ongoing research in this field continues to reveal the remarkable complexity and versatility of this seemingly simple yet profoundly important aspect of life's molecular machinery.
Latest Posts
Related Post
Thank you for visiting our website which covers about Rungs Of The Dna Ladder Made Of . We hope the information provided has been useful to you. Feel free to contact us if you have any questions or need further assistance. See you next time and don't miss to bookmark.