Oxidation State Of Carbon In C2o42
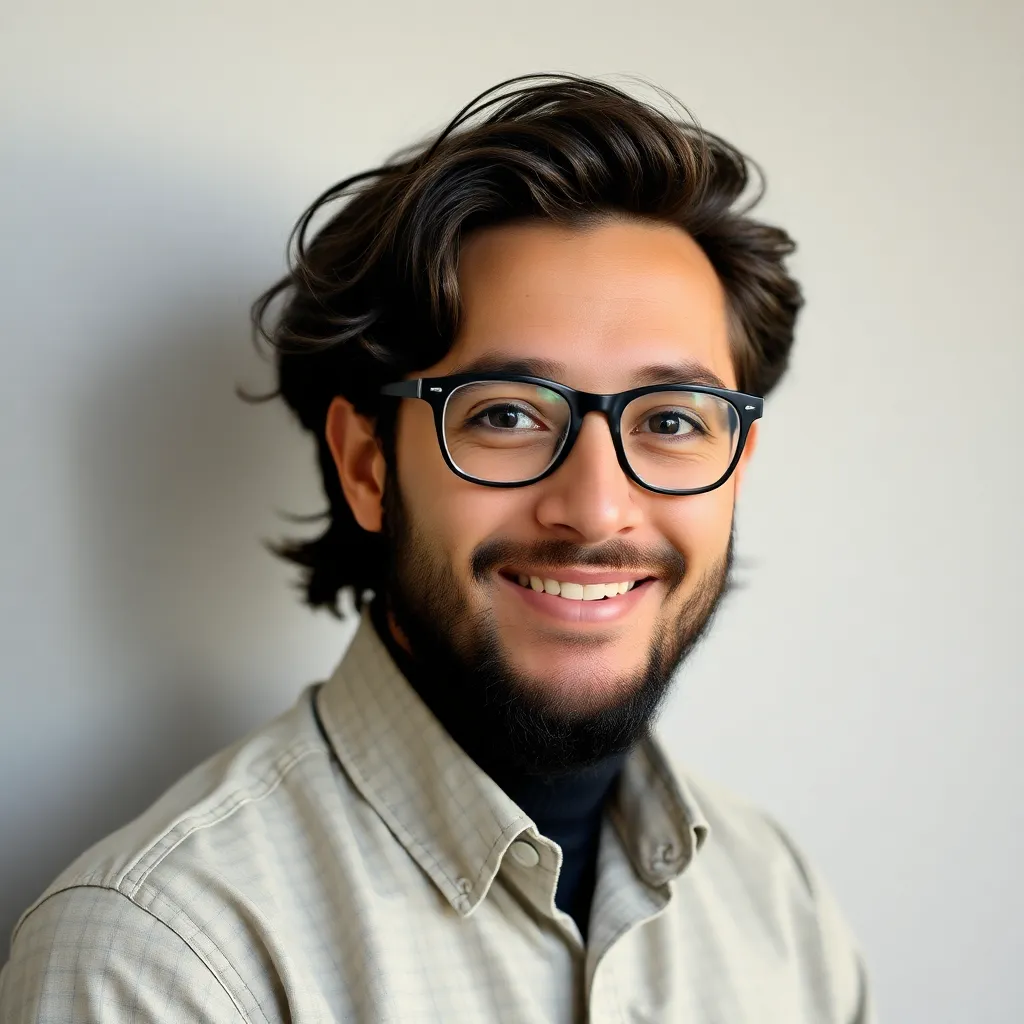
News Leon
Apr 17, 2025 · 5 min read

Table of Contents
Determining the Oxidation State of Carbon in C₂O₄²⁻ (Oxalate Ion)
The oxalate ion, C₂O₄²⁻, is a common ligand in coordination chemistry and plays a crucial role in various biochemical processes. Understanding its structure and, importantly, the oxidation state of carbon within the ion is fundamental to grasping its reactivity and behavior. This article will delve deep into the method of determining the oxidation state of carbon in C₂O₄²⁻, exploring the underlying concepts and addressing potential misconceptions.
Understanding Oxidation States
Before tackling the oxalate ion specifically, let's review the fundamental concept of oxidation states. The oxidation state, also known as the oxidation number, represents the hypothetical charge an atom would have if all bonds to atoms of different elements were completely ionic. It's a crucial tool for understanding redox reactions (reduction-oxidation reactions) and predicting the reactivity of chemical species.
Key Rules for Assigning Oxidation States:
- Free elements: The oxidation state of an atom in its elemental form is always 0 (e.g., O₂, Cl₂, C).
- Monatomic ions: The oxidation state of a monatomic ion is equal to its charge (e.g., Na⁺ = +1, Cl⁻ = -1).
- Fluorine: Fluorine always has an oxidation state of -1 in its compounds.
- Oxygen: Oxygen usually has an oxidation state of -2 in its compounds, except in peroxides (e.g., H₂O₂) where it's -1 and in superoxides (e.g., KO₂) where it's -1/2.
- Hydrogen: Hydrogen usually has an oxidation state of +1 in its compounds, except in metal hydrides (e.g., NaH) where it's -1.
- The sum of oxidation states: In a neutral molecule, the sum of the oxidation states of all atoms must be zero. In a polyatomic ion, the sum of the oxidation states must equal the charge of the ion.
Determining the Oxidation State of Carbon in C₂O₄²⁻
Now, let's apply these rules to determine the oxidation state of carbon in the oxalate ion, C₂O₄²⁻.
-
Identify the known oxidation states: We know that oxygen typically has an oxidation state of -2 (unless in a peroxide or superoxide, which isn't the case here).
-
Assign variables: Let's represent the oxidation state of carbon as 'x'. Since there are two carbon atoms in C₂O₄²⁻, the total contribution from carbon will be 2x.
-
Apply the rule of the sum of oxidation states: The oxalate ion has a charge of -2. Therefore, the sum of the oxidation states of all atoms must equal -2. This gives us the equation:
2x + 4(-2) = -2
-
Solve for x: Solving this simple algebraic equation for x, we get:
2x - 8 = -2 2x = 6 x = +3
Therefore, the oxidation state of each carbon atom in the C₂O₄²⁻ ion is +3.
Resonance Structures and Oxidation State
The oxalate ion exhibits resonance, meaning that its actual structure is a hybrid of multiple contributing Lewis structures. However, the oxidation state is not affected by resonance. Regardless of which resonance structure we consider, the overall charge distribution and the assignment of oxidation states remain consistent, resulting in a +3 oxidation state for each carbon atom.
While the delocalized electrons make it difficult to picture where a formal charge resides, using a specific Lewis structure can help clarify this calculation:
O=C-C=O
|| ||
O O
2-
In this representation (one of several resonance structures), the double bonds place the overall charge distribution to make the carbon oxidation state +3.
Implications of the +3 Oxidation State
The +3 oxidation state of carbon in the oxalate ion has several significant implications:
-
Reactivity: The relatively high oxidation state makes the oxalate ion a good reducing agent in certain redox reactions. It can donate electrons to oxidizing agents, undergoing oxidation itself.
-
Coordination Chemistry: The oxalate ion acts as a bidentate ligand, meaning it can bind to a metal ion through two oxygen atoms. The oxidation state of the carbon atoms doesn't directly participate in the coordination bond, but it influences the overall stability and electronic properties of the resulting complex.
-
Biochemistry: Oxalate is involved in various biochemical processes, including calcium oxalate formation (kidney stones) and metabolic pathways. The oxidation state of carbon is relevant in understanding its role in these processes and potential interactions with enzymes and other biomolecules.
Common Misconceptions about Oxidation States
There are several common misconceptions surrounding oxidation states that should be clarified:
-
Oxidation states are not real charges: They represent a hypothetical charge distribution based on a simplified model of electron allocation. The electrons are not fully transferred, particularly in covalent bonds.
-
Oxidation states can be fractional: In certain cases, such as in superoxides, oxidation states can have fractional values. This reflects the delocalization of electrons across multiple atoms.
-
Oxidation states are not always intuitive: Predicting oxidation states based solely on intuition can be misleading. The rules and systematic approach are essential for accurate determination.
Further Exploration of Oxalate Chemistry
The chemistry of the oxalate ion is rich and multifaceted. Further exploration could include:
-
Redox reactions involving oxalate: Examining reactions where oxalate acts as a reducing agent, and determining the products formed depending on the oxidizing agent used.
-
Coordination complexes with oxalate: Investigating the formation, structure, and properties of various metal-oxalate complexes. This involves exploring different metal ions, the stereochemistry of the complexes, and their applications in catalysis and materials science.
-
Biochemistry of oxalate metabolism: Studying the role of oxalate in biological systems, the enzymes involved in its metabolism, and the causes and consequences of oxalate accumulation (hyperoxaluria).
-
Applications of oxalate and its derivatives: Exploring the various industrial and technological applications of oxalate and its derivatives, such as in photography, electrochemistry, and the synthesis of other organic compounds.
Conclusion
The determination of the oxidation state of carbon in the oxalate ion (C₂O₄²⁻) serves as a straightforward yet illustrative example of applying fundamental principles in chemistry. The systematic approach, involving identifying known oxidation states, assigning variables, and applying the rule of the sum of oxidation states, leads to the unequivocal result of +3 for each carbon atom. Understanding this oxidation state is crucial for comprehending the reactivity, coordination chemistry, and biochemical significance of this important ion. This detailed analysis clarifies potential misconceptions and provides a solid foundation for further explorations into the fascinating world of oxalate chemistry.
Latest Posts
Latest Posts
-
The Nucleotide Chains Of Dna Are Held Together By
Apr 19, 2025
-
3 Most Common Shapes Of Bacteria
Apr 19, 2025
-
Which Is True Of Skeletal Muscles
Apr 19, 2025
-
Which Of The Following Statements Is True About Meiosis
Apr 19, 2025
-
Pepsinogen An Inactive Digestive Enzyme Is Secreted By The
Apr 19, 2025
Related Post
Thank you for visiting our website which covers about Oxidation State Of Carbon In C2o42 . We hope the information provided has been useful to you. Feel free to contact us if you have any questions or need further assistance. See you next time and don't miss to bookmark.