In The Electron Transport Chain The Final Electron Acceptor Is
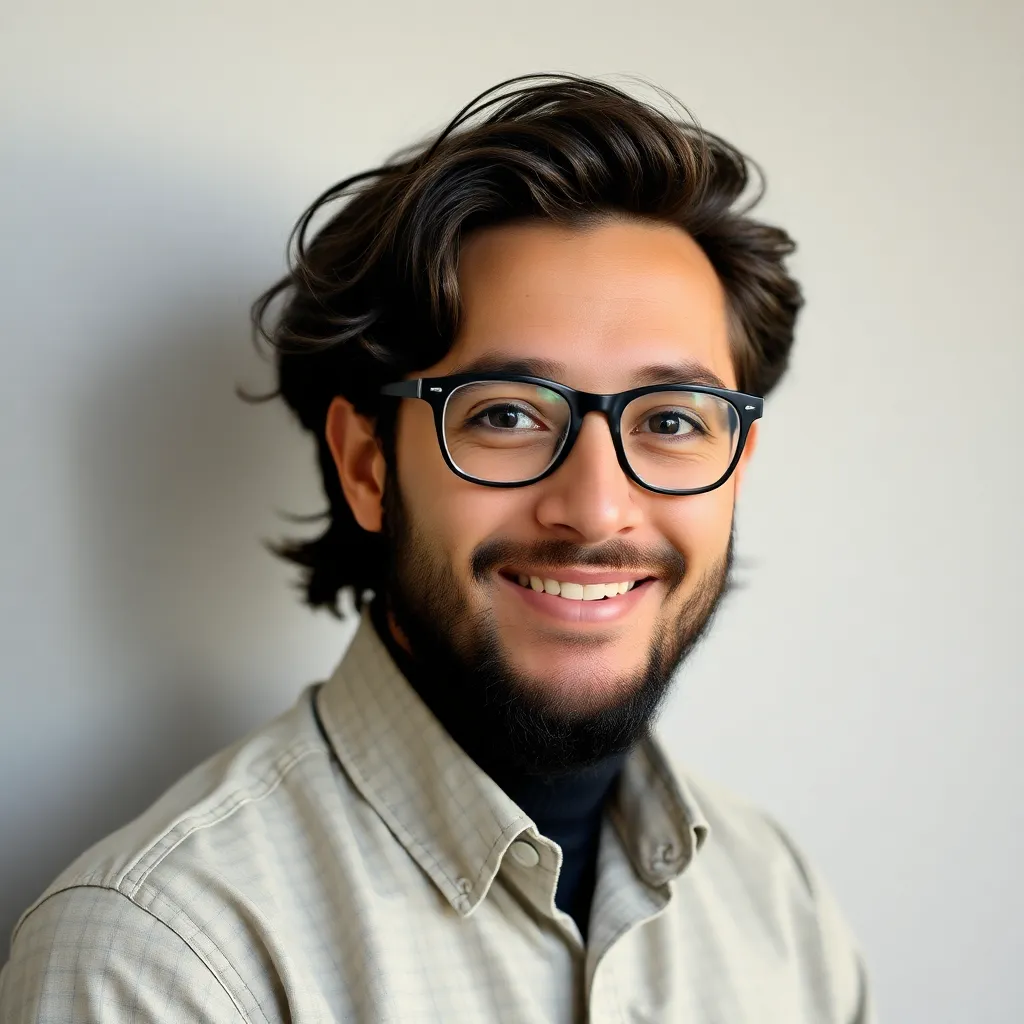
News Leon
Mar 12, 2025 · 7 min read

Table of Contents
In the Electron Transport Chain, the Final Electron Acceptor Is… Oxygen! Understanding Oxidative Phosphorylation
The electron transport chain (ETC), also known as the respiratory chain, is a fundamental process in cellular respiration, responsible for generating the majority of the ATP (adenosine triphosphate) that fuels cellular activities. Understanding this chain, and particularly the identity of the final electron acceptor, is key to grasping how our cells harvest energy from the food we eat. This article will delve deep into the ETC, exploring its components, the crucial role of the final electron acceptor, and the consequences of its absence.
The Electron Transport Chain: A Molecular Cascade of Energy Transfer
The ETC is a series of protein complexes embedded within the inner mitochondrial membrane in eukaryotes (organisms with membrane-bound organelles like mitochondria) and the plasma membrane in prokaryotes (organisms lacking such organelles). These complexes act as electron carriers, passing electrons down an energy gradient from a higher energy level to a lower one. This electron flow drives the pumping of protons (H+) across the inner mitochondrial membrane, creating a proton gradient. This gradient, in turn, fuels ATP synthesis through a process called chemiosmosis.
The Key Players: Protein Complexes and Electron Carriers
The ETC isn't a single, linear pathway, but rather a network involving several key players:
-
Complex I (NADH dehydrogenase): This complex accepts electrons from NADH (nicotinamide adenine dinucleotide), a high-energy electron carrier produced during glycolysis and the citric acid cycle. The transfer of electrons through Complex I contributes significantly to proton pumping.
-
Complex II (Succinate dehydrogenase): Unlike Complex I, Complex II accepts electrons from FADH2 (flavin adenine dinucleotide), another electron carrier produced during the citric acid cycle. Complex II contributes fewer protons to the gradient than Complex I.
-
Ubiquinone (Coenzyme Q): This mobile electron carrier shuttles electrons between Complex I or II and Complex III. Its ability to move freely within the membrane is crucial for electron transport.
-
Complex III (Cytochrome bc1 complex): This complex receives electrons from ubiquinone and passes them to cytochrome c, another mobile electron carrier. Proton pumping also occurs at this stage.
-
Cytochrome c: This small, water-soluble protein shuttles electrons from Complex III to Complex IV.
-
Complex IV (Cytochrome c oxidase): This complex receives electrons from cytochrome c and, importantly, transfers them to the final electron acceptor. This step is also associated with proton pumping.
The precise mechanism of electron transfer within each complex involves intricate redox reactions (reduction-oxidation reactions) where electrons are passed between metal ions like iron and copper, embedded within the protein structures.
The Crucial Role of the Final Electron Acceptor: Oxygen
The final electron acceptor in the electron transport chain is molecular oxygen (O₂). This is a critically important aspect of aerobic respiration. Without oxygen, the electron transport chain would cease to function effectively, leading to a significant reduction in ATP production.
The Reduction of Oxygen: Water Formation
When oxygen accepts electrons at Complex IV, it is reduced to water (H₂O). This reaction is essential for several reasons:
-
Maintaining the Electron Flow: The continuous acceptance of electrons by oxygen keeps the electron transport chain running. If oxygen isn't available, electrons accumulate within the chain, blocking further electron transfer. This buildup can lead to the production of reactive oxygen species (ROS), which are harmful to cells.
-
Generating the Proton Gradient: The reduction of oxygen is coupled to the pumping of protons across the membrane. This creates the electrochemical proton gradient that is essential for ATP synthesis. Without oxygen, the proton gradient cannot be maintained.
-
Preventing the Backflow of Electrons: The highly electronegative nature of oxygen ensures that electrons are efficiently transferred to it, preventing the reverse flow of electrons and maintaining the unidirectional flow of electrons through the chain.
The Consequences of Oxygen Absence: Anaerobic Respiration
When oxygen is absent, the electron transport chain cannot function optimally. Cells resort to anaerobic respiration, which involves alternative electron acceptors, such as nitrate, sulfate, or fumarate. These alternative pathways generate far less ATP than aerobic respiration, explaining why aerobic respiration is the preferred method of energy production in most organisms.
The switch to anaerobic respiration can have significant consequences:
-
Reduced ATP Production: The yield of ATP is significantly lower in anaerobic respiration, limiting the cell's ability to carry out energy-demanding processes.
-
Production of Fermentation Byproducts: In the absence of an external electron acceptor, cells may resort to fermentation, a metabolic process that regenerates NAD+ from NADH by producing byproducts such as lactate (in animals) or ethanol (in yeast). These byproducts can be toxic at high concentrations.
-
Cellular Damage: The buildup of electrons in the ETC in the absence of oxygen can lead to the formation of reactive oxygen species (ROS), which can damage cellular components such as DNA, proteins, and lipids.
The Importance of Oxygen in Maintaining Cellular Homeostasis
The role of oxygen as the final electron acceptor is inextricably linked to maintaining cellular homeostasis. Oxygen's high electronegativity allows for efficient electron transfer, ensuring that the ETC operates smoothly and produces sufficient ATP to power cellular processes. Its absence triggers a cascade of metabolic changes, impacting cellular function and potentially leading to cell death.
Exploring the Detailed Mechanism of Oxygen Reduction at Complex IV
Complex IV, also known as cytochrome c oxidase, is a complex transmembrane protein with a crucial role in the final step of the electron transport chain. Its active site contains copper ions and heme groups that facilitate the reduction of molecular oxygen to water. The process involves a series of intricate steps:
-
Electron Transfer: Four electrons are sequentially transferred from cytochrome c to the copper ions and heme groups within Complex IV.
-
Oxygen Binding: Molecular oxygen (O₂) binds to the active site.
-
Reduction to Water: The four electrons reduce the bound oxygen molecule to two molecules of water (2H₂O).
-
Proton Pumping: The reduction of oxygen is coupled to the pumping of protons across the mitochondrial membrane. This step contributes significantly to the establishment of the proton gradient that drives ATP synthesis.
-
Water Release: The newly formed water molecules are released.
The precise mechanism of oxygen reduction involves complex interactions between the metal ions, the oxygen molecule, and the surrounding protein structure. The efficiency of this process is crucial for maintaining the function of the electron transport chain and the overall energy production of the cell. Any disruption in this process can lead to a variety of metabolic defects.
Clinical Significance and Disorders Affecting the Electron Transport Chain
Disruptions in the electron transport chain can have significant clinical implications, leading to a range of mitochondrial disorders. These disorders can manifest in various ways depending on the specific affected complex and the severity of the defect. Some common clinical manifestations include:
-
Myopathy: Muscle weakness and fatigue are common symptoms due to impaired energy production in muscle cells.
-
Encephalopathy: Neurological symptoms, including seizures, developmental delays, and cognitive impairment, can occur due to impaired energy production in brain cells.
-
Cardiomyopathy: Heart muscle dysfunction can result from impaired energy production in cardiac muscle cells.
-
Lactic Acidosis: A buildup of lactate due to reduced ATP production and reliance on anaerobic metabolism.
-
Multi-systemic involvement: In severe cases, multiple organ systems can be affected, leading to severe health complications.
These disorders often have genetic origins, with mutations affecting genes encoding components of the electron transport chain complexes. Diagnosing these disorders can be challenging, often requiring specialized testing, such as biochemical assays measuring mitochondrial enzyme activities and genetic analysis identifying mutations in relevant genes.
Conclusion: The Unsung Hero of Cellular Respiration
The final electron acceptor in the electron transport chain, oxygen, is an unsung hero of cellular respiration. Its ability to accept electrons efficiently enables the generation of a large amount of ATP, powering cellular processes and maintaining cellular homeostasis. Understanding the intricate mechanism of the electron transport chain, the critical role of oxygen, and the consequences of its absence is crucial for appreciating the fundamental processes of life and the implications of disruptions in this essential metabolic pathway. The impact extends beyond basic cell biology, highlighting the importance of this pathway in human health and disease. Further research continues to unravel the complexities of this remarkable system, offering the potential for future therapies targeting mitochondrial dysfunction.
Latest Posts
Related Post
Thank you for visiting our website which covers about In The Electron Transport Chain The Final Electron Acceptor Is . We hope the information provided has been useful to you. Feel free to contact us if you have any questions or need further assistance. See you next time and don't miss to bookmark.