Ground State Electron Configuration For F2
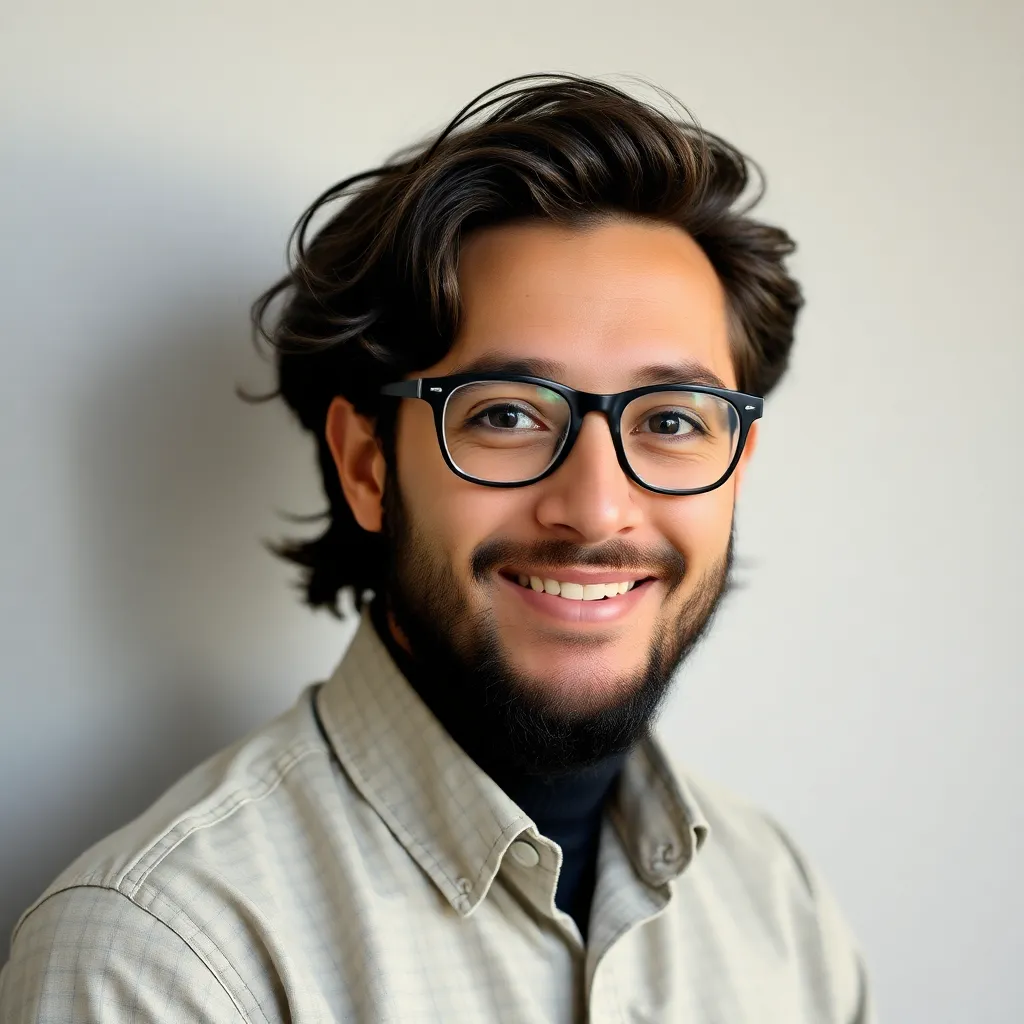
News Leon
Apr 11, 2025 · 5 min read

Table of Contents
Ground State Electron Configuration for F₂: A Deep Dive into Molecular Orbital Theory
The determination of the ground state electron configuration for diatomic fluorine, F₂, provides a fascinating case study in molecular orbital (MO) theory. Unlike simpler diatomic molecules like H₂ or O₂, F₂'s electron configuration exhibits nuances that demand a thorough understanding of bonding and antibonding orbitals, orbital hybridization, and the principles governing electron filling. This article delves into the intricacies of F₂'s electron configuration, explaining the underlying theoretical framework and providing a step-by-step guide to its derivation.
Understanding Molecular Orbital Theory
Before diving into F₂'s specifics, let's establish a foundational understanding of molecular orbital theory. This theory postulates that when atoms combine to form a molecule, their atomic orbitals combine to create new molecular orbitals. These molecular orbitals encompass the entire molecule, not just individual atoms. Crucially, the number of molecular orbitals formed always equals the number of atomic orbitals that combined.
Bonding and Antibonding Orbitals
Molecular orbitals are classified into two main types: bonding and antibonding. Bonding orbitals are lower in energy than the original atomic orbitals and concentrate electron density between the nuclei, thus strengthening the bond. Antibonding orbitals, on the other hand, are higher in energy and have electron density concentrated outside the region between the nuclei, thus weakening the bond or even destabilizing the molecule.
Linear Combination of Atomic Orbitals (LCAO)
The mathematical description of molecular orbital formation involves the linear combination of atomic orbitals (LCAO). Essentially, this method combines the wave functions of atomic orbitals to generate molecular orbital wave functions. The resulting molecular orbitals are often represented as linear combinations of the atomic orbitals involved.
Constructing the Molecular Orbitals of F₂
Fluorine (F) has an atomic number of 9, with an electron configuration of 1s²2s²2p⁵. When two fluorine atoms approach each other to form F₂, their atomic orbitals interact to form molecular orbitals. The relevant orbitals for bonding are the 2s and 2p orbitals.
Sigma (σ) and Pi (π) Orbitals
The interaction between atomic orbitals leads to the formation of both sigma (σ) and pi (π) molecular orbitals. Sigma (σ) orbitals are formed by the head-on overlap of atomic orbitals, resulting in cylindrical symmetry around the internuclear axis. Pi (π) orbitals are formed by the side-on overlap of atomic orbitals, resulting in a nodal plane containing the internuclear axis.
In the case of F₂, the 2s atomic orbitals of each fluorine atom combine to form one bonding σ<sub>2s</sub> and one antibonding σ*<sub>2s</sub> molecular orbital. Similarly, the 2p orbitals interact to form both σ and π molecular orbitals. The 2p<sub>z</sub> orbitals (where z is the internuclear axis) combine to form a σ<sub>2pz</sub> bonding and a σ*<sub>2pz</sub> antibonding molecular orbital. The 2p<sub>x</sub> and 2p<sub>y</sub> orbitals combine to form two sets of degenerate π<sub>2px</sub>, π<sub>2py</sub> bonding and π*<sub>2px</sub>, π*<sub>2py</sub> antibonding molecular orbitals.
Filling the Molecular Orbitals: The Electron Configuration of F₂
Now that we've established the molecular orbitals, let's populate them with electrons. Each fluorine atom contributes 7 valence electrons (2s²2p⁵), so the F₂ molecule has a total of 14 valence electrons. These electrons are filled into the molecular orbitals according to the Aufbau principle and Hund's rule, with lower energy orbitals filled first, and electrons occupying degenerate orbitals singly before pairing up.
The order of increasing energy for the molecular orbitals in F₂ is typically: σ<sub>2s</sub> < σ*<sub>2s</sub> < σ<sub>2pz</sub> < π<sub>2px</sub> = π<sub>2py</sub> < π*<sub>2px</sub> = π*<sub>2py</sub> < σ*<sub>2pz</sub>. Note that the exact order can vary slightly depending on the level of approximation used in the calculations.
Following this order, the 14 valence electrons are distributed as follows:
- σ<sub>2s</sub>: 2 electrons
- σ*<sub>2s</sub>: 2 electrons
- σ<sub>2pz</sub>: 2 electrons
- π<sub>2px</sub>: 2 electrons
- π<sub>2py</sub>: 2 electrons
- π*<sub>2px</sub>: 2 electrons
- π*<sub>2py</sub>: 2 electrons
- σ*<sub>2pz</sub>: 0 electrons
Therefore, the ground state electron configuration for F₂ is: (σ<sub>2s</sub>)²(σ*<sub>2s</sub>)²(σ<sub>2pz</sub>)²(π<sub>2px</sub>)²(π<sub>2py</sub>)²(π*<sub>2px</sub>)²(π*<sub>2py</sub>)²
Bond Order and Stability
The bond order is a crucial indicator of the strength and stability of a chemical bond. It is calculated as half the difference between the number of electrons in bonding orbitals and the number of electrons in antibonding orbitals.
For F₂, the bond order is: (8 - 6) / 2 = 1
A bond order of 1 indicates a single covalent bond between the two fluorine atoms. This explains the observed stability of the F₂ molecule.
Comparing F₂ to Other Diatomic Molecules
It's instructive to compare the electron configuration and bonding in F₂ to other diatomic molecules of the second period. For instance, O₂ (with 12 valence electrons) has a bond order of 2 (a double bond), while N₂ (with 10 valence electrons) boasts a triple bond (bond order 3). The presence of electrons in antibonding orbitals in F₂ weakens the bond compared to O₂ and N₂, illustrating the importance of antibonding orbitals in determining molecular properties.
Advanced Considerations and Computational Methods
While the LCAO-MO approach provides a valuable conceptual framework, more sophisticated methods are necessary for accurate predictions of molecular properties. These methods include Hartree-Fock calculations and density functional theory (DFT), which incorporate electron correlation and provide more refined depictions of molecular orbitals and electronic structure. These advanced computational techniques can provide more precise energy levels and orbital shapes.
Conclusion
Determining the ground state electron configuration for F₂ involves applying the principles of molecular orbital theory. Understanding the formation of bonding and antibonding orbitals, the linear combination of atomic orbitals, and the filling of orbitals according to the Aufbau principle and Hund's rule is essential. The resulting electron configuration explains the observed single bond in F₂, a stability arising from the balance between bonding and antibonding electrons. The discussion also highlights the need for more advanced computational methods to achieve greater accuracy in predicting molecular properties. While the simple model provided here is insightful, remember that the true electron distribution is far more complex, reflecting the nuanced quantum mechanical nature of molecular bonding.
Latest Posts
Latest Posts
-
Current Cannot Flow Through A Circuit When The Switch Is
Apr 18, 2025
-
Find The Measure Of Angle Bcd
Apr 18, 2025
-
Provincial Governor Of The Mughal Empire
Apr 18, 2025
-
Which Functional Group Can Act As An Acid
Apr 18, 2025
-
64 As A Fraction In Simplest Form
Apr 18, 2025
Related Post
Thank you for visiting our website which covers about Ground State Electron Configuration For F2 . We hope the information provided has been useful to you. Feel free to contact us if you have any questions or need further assistance. See you next time and don't miss to bookmark.