Eukaryotic Chromatin Is Composed Of Which Of The Following Macromolecules
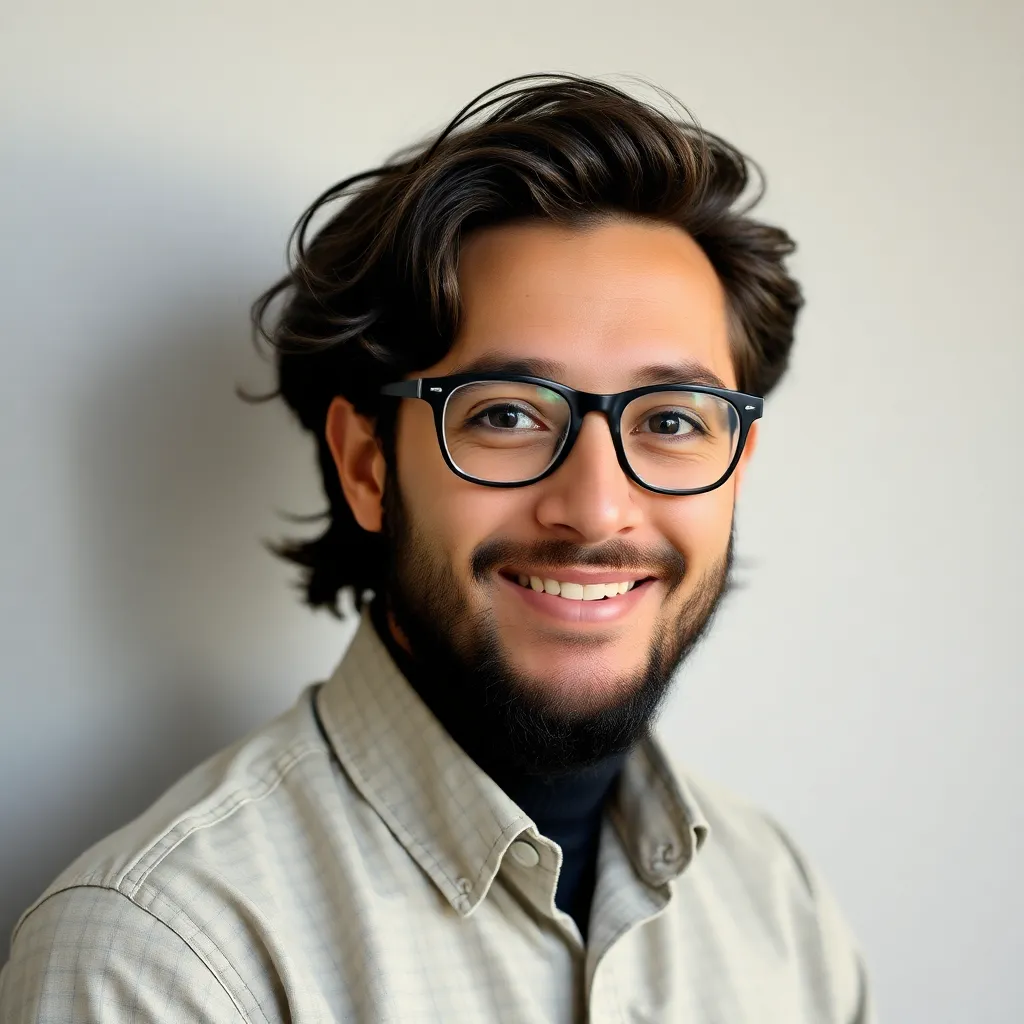
News Leon
Apr 17, 2025 · 6 min read

Table of Contents
Eukaryotic Chromatin: A Deep Dive into its Macromolecular Composition
Eukaryotic chromatin, the fundamental building block of eukaryotic chromosomes, is a complex and dynamic structure crucial for organizing and regulating the genome. Understanding its composition is key to comprehending numerous cellular processes, including DNA replication, transcription, repair, and cell division. This article delves into the macromolecules that constitute eukaryotic chromatin, exploring their individual roles and their intricate interplay in shaping chromosome structure and function.
The Core Components: DNA, Histones, and Non-Histone Proteins
Eukaryotic chromatin is primarily composed of three major classes of macromolecules:
-
Deoxyribonucleic Acid (DNA): The genetic blueprint of the cell, DNA carries the hereditary information that directs all cellular activities. In chromatin, DNA is tightly packaged and organized to fit within the confines of the nucleus. Its linear sequence dictates the order of amino acids in proteins, ultimately determining an organism’s traits and functions. The immense length of DNA necessitates a highly efficient packaging mechanism, achieved through its interaction with histones and other proteins.
-
Histone Proteins: These are highly conserved basic proteins, positively charged due to a high abundance of lysine and arginine residues. This positive charge allows them to interact strongly with the negatively charged DNA phosphate backbone. Histones are the fundamental structural components of chromatin, forming the nucleosomes, the basic units of chromatin organization. There are five main histone families: H1, H2A, H2B, H3, and H4. H2A, H2B, H3, and H4 form the histone octamer, the core around which DNA is wrapped. H1 is a linker histone, binding to the linker DNA between nucleosomes and contributing to higher-order chromatin structure.
-
Non-Histone Proteins: This diverse group encompasses a vast array of proteins with various functions in chromatin structure and regulation. They include:
-
Chromatin Remodeling Complexes: These multi-protein complexes use ATP hydrolysis to alter the position and conformation of nucleosomes, making DNA more or less accessible to regulatory proteins. This dynamic remodeling plays a vital role in gene regulation.
-
Transcription Factors: These proteins bind to specific DNA sequences and regulate gene expression by either promoting or inhibiting transcription. Their interaction with chromatin is crucial for controlling which genes are expressed at a given time.
-
DNA Replication and Repair Proteins: These proteins are essential for maintaining genome integrity. They facilitate DNA replication during cell division and repair DNA damage, preventing mutations and chromosomal instability. Their access to DNA requires a regulated interaction with the chromatin structure.
-
Structural Proteins: These proteins contribute to the higher-order organization of chromatin, forming the chromosome scaffold and contributing to chromosome condensation during mitosis and meiosis.
-
Nucleosome: The Fundamental Unit of Chromatin
The fundamental repeating unit of chromatin is the nucleosome. A nucleosome comprises approximately 147 base pairs of DNA wrapped around a histone octamer, consisting of two copies each of H2A, H2B, H3, and H4 histones. This wrapping is crucial for compacting the DNA. The DNA wraps around the octamer approximately 1.65 times, forming a left-handed superhelix. The linker DNA, varying in length depending on the organism and cell type, connects adjacent nucleosomes. H1 histone binds to the linker DNA and contributes to the higher-order folding of chromatin.
Histone Modifications: A Dynamic Regulatory Code
Histones are not static structures; they are subject to a wide range of post-translational modifications, including:
-
Acetylation: Addition of acetyl groups to lysine residues, usually resulting in relaxed chromatin structure and increased gene transcription. Histone acetyltransferases (HATs) add acetyl groups, while histone deacetylases (HDACs) remove them.
-
Methylation: Addition of methyl groups to lysine or arginine residues. Methylation can have diverse effects on gene expression depending on the specific residue and the number of methyl groups added. Histone methyltransferases (HMTs) add methyl groups, while histone demethylases remove them.
-
Phosphorylation: Addition of phosphate groups to serine or threonine residues. Phosphorylation is often associated with chromosome condensation and mitotic processes.
-
Ubiquitination: Addition of ubiquitin, a small protein, to lysine residues. Ubiquitination can have diverse effects depending on the specific lysine residue and the context.
-
Sumoylation: Attachment of small ubiquitin-related modifier (SUMO) proteins. Sumoylation can influence chromatin structure and gene expression.
These modifications act as a histone code, influencing chromatin structure and regulating gene expression. The combination and location of these modifications create a complex regulatory landscape that dynamically influences genome function.
Higher-Order Chromatin Structure: From Nucleosomes to Chromosomes
The nucleosomes are not randomly arranged but are organized into higher-order structures. The arrangement of nucleosomes into 30nm fibers is a crucial step in DNA compaction, reducing its length by a factor of 6. The exact structure of the 30nm fiber remains a subject of ongoing research, with models proposing various arrangements, including zigzag and solenoid structures.
Further compaction leads to the formation of chromatin loops, which are anchored to a protein scaffold. These loops contribute to the organization of chromosomes into distinct functional domains. During mitosis and meiosis, chromosomes undergo dramatic condensation, reaching a highly compacted state that facilitates segregation of sister chromatids. This condensation involves a complex interplay of various proteins, including condensins and cohesins.
The Role of Non-Histone Proteins in Chromatin Structure and Function
Non-histone proteins are crucial for various aspects of chromatin function, including:
-
Chromatin Remodeling: Proteins like SWI/SNF complexes use ATP hydrolysis to reposition nucleosomes, making DNA more or less accessible to the transcriptional machinery. This remodeling is vital for regulating gene expression.
-
Gene Regulation: Transcription factors bind to specific DNA sequences to regulate the expression of nearby genes. Their access to DNA is often controlled by chromatin structure and modifications.
-
DNA Replication and Repair: Proteins involved in DNA replication and repair must access the DNA template, often requiring alterations in chromatin structure.
-
Chromosome Segregation: Proteins like condensins and cohesins play crucial roles in chromosome condensation and segregation during cell division.
Chromatin Dynamics and its Implications
Chromatin is not a static structure; it's highly dynamic, undergoing continuous remodeling to adapt to cellular needs. Changes in chromatin structure, often involving histone modifications and nucleosome repositioning, are crucial for various cellular processes, including:
-
Gene Regulation: Dynamic changes in chromatin accessibility control which genes are transcribed and at what level. This precise control is essential for cellular differentiation, development, and response to environmental stimuli.
-
DNA Replication: Chromatin must be properly reorganized to allow access to the DNA replication machinery.
-
DNA Repair: Chromatin structure must be altered to allow access to the DNA repair machinery to fix DNA damage.
-
Cell Cycle Progression: Chromatin undergoes dramatic changes during the cell cycle, with condensation and decondensation events coordinating chromosome segregation and cell division.
Conclusion: The Intricate Dance of Macromolecules in Eukaryotic Chromatin
Eukaryotic chromatin is a sophisticated and intricately organized structure, built from the interplay of DNA, histones, and a multitude of non-histone proteins. Its dynamic nature, governed by histone modifications and chromatin remodeling, is critical for regulating gene expression, maintaining genome integrity, and orchestrating crucial cellular processes. Understanding the molecular composition and dynamic behavior of chromatin remains a central focus in biological research, with significant implications for understanding human health and disease. Future research will undoubtedly continue to refine our understanding of this fascinating and vital cellular component. Further research is needed to fully elucidate the intricate mechanisms governing chromatin structure and function, paving the way for potential therapeutic interventions for various diseases associated with chromatin dysfunction. The remarkable complexity of chromatin serves as a testament to the elegance and efficiency of biological systems.
Latest Posts
Latest Posts
-
What Is The Perimeter Of Defg
Apr 19, 2025
-
How Many Lines Of Symmetry Does An Isosceles Trapezoid Have
Apr 19, 2025
-
What Percent Of An Hour Is 45 Minutes
Apr 19, 2025
-
Which Material Cannot Be Made Into A Magnet
Apr 19, 2025
-
What Is The Net Gain Of Atp From Glycolysis
Apr 19, 2025
Related Post
Thank you for visiting our website which covers about Eukaryotic Chromatin Is Composed Of Which Of The Following Macromolecules . We hope the information provided has been useful to you. Feel free to contact us if you have any questions or need further assistance. See you next time and don't miss to bookmark.