Electronic Configuration Of Copper And Chromium
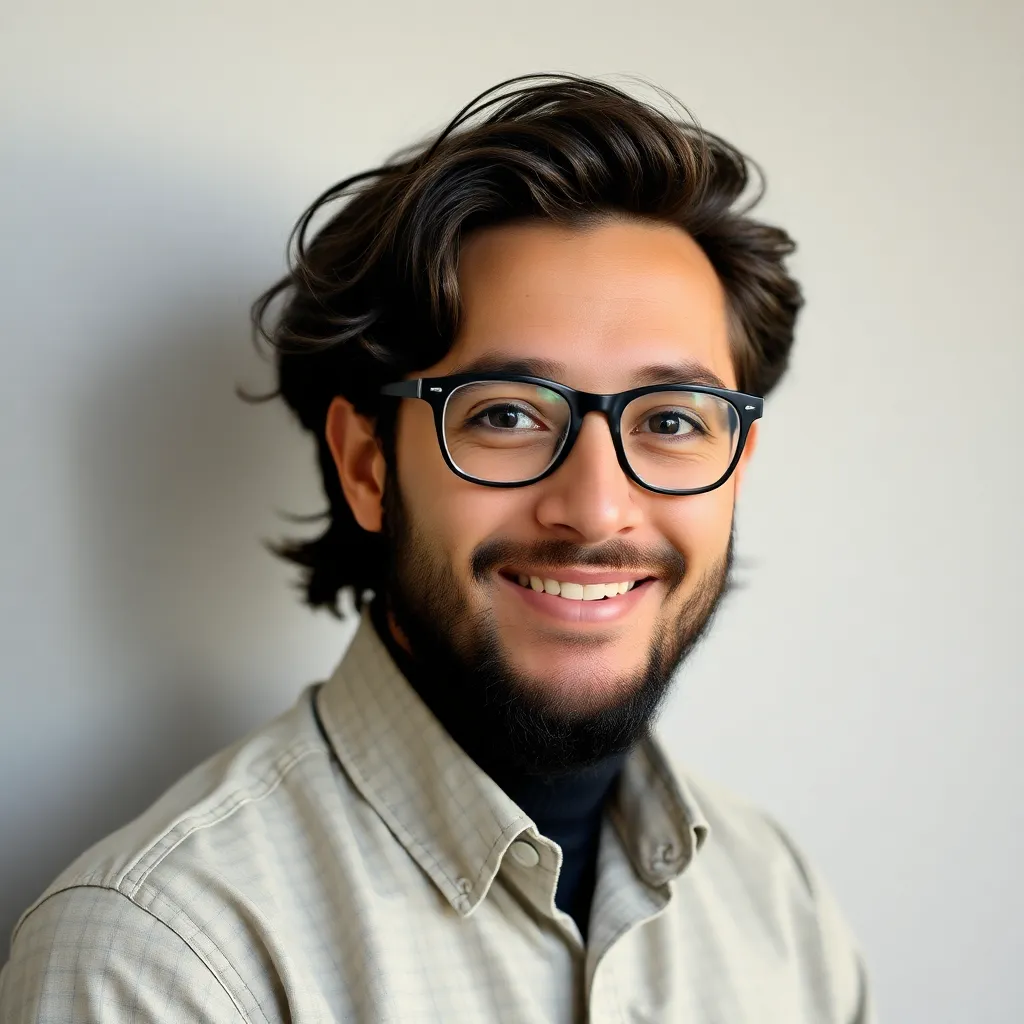
News Leon
Apr 14, 2025 · 5 min read

Table of Contents
The Exceptional Electronic Configurations of Copper and Chromium: A Deep Dive
The periodic table, a cornerstone of chemistry, organizes elements based on their atomic structure and properties. While the Aufbau principle and Hund's rule generally predict electronic configurations, certain elements, notably copper (Cu) and chromium (Cr), exhibit exceptions. Understanding these exceptions requires delving into the intricacies of electron-electron interactions and orbital stability. This article explores the electronic configurations of copper and chromium, examining the underlying reasons for their deviations from expected patterns and the consequences of these anomalies.
Understanding Electronic Configuration
Before delving into the exceptions, let's establish a foundational understanding of electronic configuration. The electronic configuration of an atom describes how electrons are distributed among its various energy levels (shells) and sublevels (subshells). These are represented using a notation that indicates the principal quantum number (n), the azimuthal quantum number (l), and the number of electrons in each subshell. For instance, 1s² signifies two electrons in the 1s subshell.
The Aufbau principle, which dictates that electrons fill orbitals starting with the lowest energy level, is generally followed. Hund's rule further states that electrons will individually occupy orbitals within a subshell before pairing up. These rules provide a framework for predicting electronic configurations, but they aren't absolute.
The Expected Electronic Configurations of Copper and Chromium
Based on the Aufbau principle and Hund's rule, we might expect copper (atomic number 29) to have an electronic configuration of [Ar] 3d⁹ 4s². Similarly, chromium (atomic number 24) should have a configuration of [Ar] 3d⁴ 4s². Here, [Ar] represents the electron configuration of Argon, a noble gas with 18 electrons.
However, experimental evidence reveals that these predictions are incorrect.
The Actual Electronic Configurations: The Exceptions
The actual electronic configuration of copper is [Ar] 3d¹⁰ 4s¹, and for chromium, it's [Ar] 3d⁵ 4s¹. These deviations from the expected configurations present a fascinating anomaly that requires explanation.
Why the Exceptions? The Role of Exchange Energy and Orbital Stability
The key to understanding these exceptions lies in the concept of exchange energy. Exchange energy is a quantum mechanical phenomenon that arises from the interaction between electrons with parallel spins. Electrons with parallel spins tend to repel each other less strongly than electrons with opposite spins. This reduced repulsion leads to a decrease in the overall energy of the system.
In the case of chromium, having a half-filled 3d subshell (3d⁵) and a single electron in the 4s subshell provides significant exchange energy stabilization. The energy gained from this exchange energy outweighs the energy cost associated with promoting an electron from the 3d to the 4s subshell. A completely filled or half-filled subshell provides extra stability because of maximized exchange energy and symmetrical electron distribution.
Similarly, for copper, a completely filled 3d subshell (3d¹⁰) results in maximum exchange energy and enhanced orbital stability. This configuration is more energetically favorable than the expected [Ar] 3d⁹ 4s² configuration, even though it involves promoting an electron from the 4s to the 3d subshell. The energy gain from achieving a completely filled d-subshell outweighs the loss associated with this electron promotion.
Inter-electronic Repulsion and Shielding Effects
The stability gained through exchange energy is also related to inter-electronic repulsion and shielding effects. Electrons in the same subshell experience stronger repulsion than electrons in different subshells. In the expected configuration of copper ([Ar] 3d⁹ 4s²), the electron in the 4s orbital experiences less effective shielding from the nuclear charge compared to the electrons in the 3d orbitals. This enhanced repulsion contributes to the higher energy of this configuration. The fully filled 3d¹⁰ subshell in the actual configuration offers better shielding, resulting in lower repulsion and improved stability.
Spectroscopic Evidence Supporting the Exceptions
The observed electronic configurations of copper and chromium are not mere theoretical predictions; they're confirmed through experimental spectroscopic data. Techniques like photoelectron spectroscopy and X-ray photoelectron spectroscopy provide direct evidence of the electron distribution, confirming the presence of a fully filled 3d subshell in copper and a half-filled 3d subshell in chromium. These experimental findings corroborate the theoretical explanations based on exchange energy and orbital stability.
Consequences of the Exceptional Electronic Configurations
The exceptional electronic configurations of copper and chromium have consequences for their chemical and physical properties. For example, the stability of the filled d-subshell in copper influences its oxidation states and its tendency to form stable complexes. Chromium’s half-filled d-subshell contributes to its diverse oxidation states and its ability to form colorful compounds. The distinctive properties of these elements arise directly from their unusual electron configurations.
Other Exceptions: Beyond Copper and Chromium
While copper and chromium are the most well-known examples, other elements also exhibit exceptions to the Aufbau principle. These exceptions are less common but highlight the complexities of electron-electron interactions within the atom. The relative energies of the subshells are affected by various factors including electron-electron repulsion, shielding effects, and penetration effects. These nuances make it challenging to always predict electron configurations simply based on the Aufbau principle alone.
Practical Applications and Relevance
Understanding the electronic configuration of elements is fundamental to numerous fields. It's crucial in:
- Chemistry: Predicting reactivity, oxidation states, and the formation of chemical bonds.
- Materials Science: Designing materials with specific properties, such as conductivity or magnetism.
- Physics: Understanding atomic spectra and the behavior of atoms in various environments.
- Spectroscopy: Interpreting spectroscopic data and analyzing the composition of materials.
Conclusion: The Intricacies of Atomic Structure
The exceptional electronic configurations of copper and chromium offer valuable insights into the complexities of atomic structure. The deviations from the expected Aufbau principle highlight the importance of considering factors like exchange energy, orbital stability, and inter-electronic repulsion in determining electron distribution. Understanding these exceptions is essential for a comprehensive understanding of chemical behavior and properties, emphasizing that the rules are guidelines, not absolutes, within the fascinating world of atomic structure. The study of these anomalies contributes to the ongoing evolution of our understanding of quantum mechanics and the behavior of matter at the atomic level. While the Aufbau principle serves as a useful starting point, it’s the subtle interplay of various quantum mechanical phenomena that ultimately dictates the electronic configuration of an atom, providing us with a deeper appreciation of the intricate elegance governing the universe's fundamental building blocks.
Latest Posts
Related Post
Thank you for visiting our website which covers about Electronic Configuration Of Copper And Chromium . We hope the information provided has been useful to you. Feel free to contact us if you have any questions or need further assistance. See you next time and don't miss to bookmark.