Carriers Transport Solutes Across The Plasma Membrane By
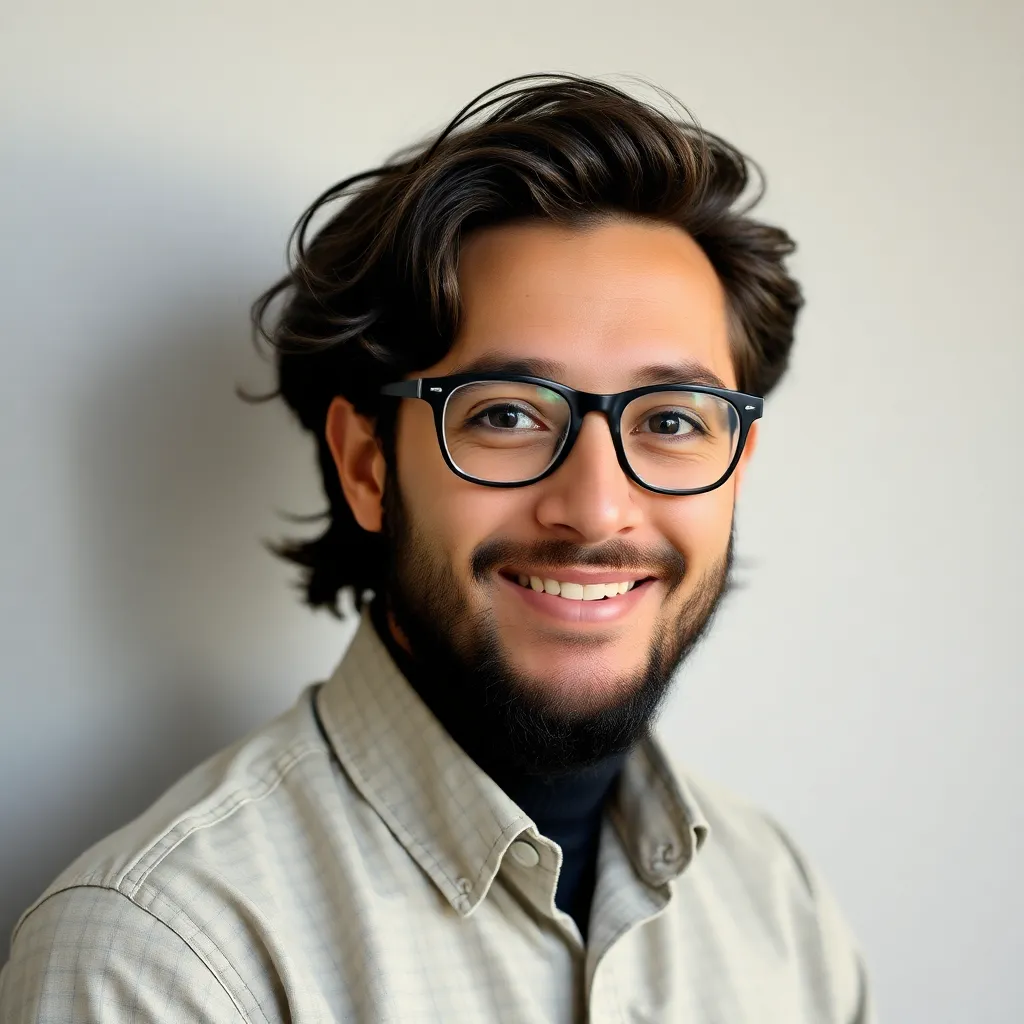
News Leon
Apr 08, 2025 · 6 min read

Table of Contents
Carriers Transport Solutes Across the Plasma Membrane By: Facilitated Diffusion and Active Transport
The plasma membrane, a selectively permeable barrier, regulates the passage of substances into and out of the cell. While small, nonpolar molecules can diffuse freely across the lipid bilayer, larger or polar molecules require assistance from membrane proteins. Among these proteins, carriers play a crucial role in transporting solutes across the membrane via two primary mechanisms: facilitated diffusion and active transport. This article will delve deep into these processes, exploring their mechanisms, energetics, and significance in cellular function.
Facilitated Diffusion: Passive Transport with Carrier Assistance
Facilitated diffusion, a type of passive transport, utilizes carrier proteins to facilitate the movement of solutes across the membrane down their concentration gradient. This means the solute moves from an area of high concentration to an area of low concentration, a process that doesn't require energy input from the cell. The carrier protein acts as a facilitator, speeding up the process compared to simple diffusion.
The Mechanism of Facilitated Diffusion
-
Binding: The solute molecule binds to a specific site on the carrier protein. The binding site's specificity ensures that only the correct solute molecule can interact with the carrier. This high specificity is a key feature differentiating facilitated diffusion from simple diffusion.
-
Conformational Change: Upon binding, the carrier protein undergoes a conformational change. This change in shape alters the protein's orientation within the membrane, exposing the solute-binding site to the opposite side of the membrane.
-
Release: The solute is released from the carrier protein on the other side of the membrane. The concentration gradient drives this release; the lower concentration on the receiving side ensures that the solute is less likely to rebind.
-
Return to Original Conformation: After the solute is released, the carrier protein returns to its original conformation, ready to facilitate the transport of another solute molecule.
Examples of Facilitated Diffusion
Many important molecules utilize facilitated diffusion, including:
-
Glucose: Glucose transporters (GLUTs) are a family of carrier proteins that facilitate glucose uptake into cells. Different GLUT isoforms have varying affinities for glucose and are expressed in different tissues. This allows for precise regulation of glucose uptake depending on the cellular needs.
-
Amino Acids: Various carrier proteins facilitate the transport of different amino acids across the plasma membrane. These transporters often exhibit high specificity for particular amino acids or groups of amino acids.
-
Ions: While some ions can passively diffuse through ion channels, others rely on facilitated diffusion via carrier proteins. These carrier proteins often show selectivity for specific ions, ensuring controlled ion movement.
Kinetics of Facilitated Diffusion
Unlike simple diffusion, facilitated diffusion exhibits saturation kinetics. At high solute concentrations, the rate of transport plateaus as all the carrier proteins become occupied. This saturation point is a characteristic feature easily distinguishing facilitated diffusion from simple diffusion.
Active Transport: Moving Solutes Against the Concentration Gradient
Active transport, in contrast to facilitated diffusion, moves solutes against their concentration gradient, from an area of low concentration to an area of high concentration. This process requires energy input, typically in the form of ATP hydrolysis.
Primary Active Transport: Directly Coupled to ATP Hydrolysis
Primary active transport directly utilizes ATP hydrolysis to power the movement of solutes. The most prominent example is the sodium-potassium pump (Na+/K+ ATPase).
The Sodium-Potassium Pump (Na+/K+ ATPase): A Detailed Look
-
Binding: Three sodium ions (Na+) bind to the intracellular sites on the pump.
-
Phosphorylation: ATP hydrolysis provides the energy for phosphorylation of the pump protein. This phosphorylation induces a conformational change.
-
Conformational Change & Release: The conformational change exposes the Na+ binding sites to the extracellular side, leading to their release.
-
Potassium Binding: Two potassium ions (K+) bind to extracellular sites on the pump.
-
Dephosphorylation: Dephosphorylation of the pump protein triggers another conformational change.
-
K+ Release: The conformational change exposes the K+ binding sites to the intracellular side, leading to their release. The pump is now back in its original conformation, ready to repeat the cycle.
The Na+/K+ pump plays a crucial role in maintaining the electrochemical gradient across the plasma membrane, which is essential for various cellular processes, including nerve impulse transmission and muscle contraction. Its function is vital for maintaining cell volume and also for secondary active transport.
Secondary Active Transport: Indirectly Coupled to ATP Hydrolysis
Secondary active transport uses the energy stored in an electrochemical gradient, established by primary active transport (like the Na+/K+ pump), to move another solute against its concentration gradient. This gradient acts as an energy source, indirectly driving the transport.
Symport and Antiport: Two Types of Secondary Active Transport
-
Symport: In symport, two solutes move across the membrane in the same direction. For example, the sodium-glucose cotransporter (SGLT) uses the energy stored in the sodium gradient to transport glucose against its concentration gradient. As sodium moves down its concentration gradient into the cell, it drags glucose along with it.
-
Antiport: In antiport, two solutes move across the membrane in opposite directions. The sodium-calcium exchanger (NCX) is an example of an antiport. It uses the inward movement of sodium ions to drive the outward movement of calcium ions. This mechanism is essential for maintaining low intracellular calcium levels, crucial for muscle relaxation and other cellular functions.
Carrier Protein Structure and Function
Carrier proteins are integral membrane proteins, meaning they are embedded within the lipid bilayer. Their structure is diverse, reflecting their varied roles in transporting different solutes.
Many carrier proteins are composed of multiple transmembrane domains, α-helices that span the lipid bilayer. These α-helices form a channel or binding site for the solute. The specific amino acid sequence within these domains determines the protein's selectivity and affinity for the solute.
Regulation of Carrier Protein Activity
The activity of carrier proteins can be regulated in several ways:
-
Hormonal Regulation: Hormones can influence carrier protein activity by altering their expression levels, their conformation, or their interaction with other proteins. For instance, insulin stimulates glucose uptake by increasing the activity of GLUT4 transporters.
-
Allosteric Regulation: Binding of a molecule to a site other than the solute-binding site (allosteric site) can modulate carrier protein activity. This allows for fine-tuning of transport in response to cellular needs.
-
Phosphorylation: Phosphorylation of carrier proteins can alter their conformation and activity. This regulatory mechanism is commonly involved in cellular signaling pathways.
Clinical Significance of Carrier-Mediated Transport
Dysfunction of carrier proteins can lead to various diseases. For example:
-
Glucose-Galactose Malabsorption: A genetic disorder characterized by defects in a sodium-glucose cotransporter (SGLT1), leading to severe diarrhea and malabsorption of glucose and galactose.
-
Cystinuria: A genetic defect affecting amino acid transport, leading to the formation of cystine stones in the kidneys.
-
Familial Hypercholesterolemia: A genetic disorder causing elevated cholesterol levels in the blood due to impaired function of LDL receptors, a type of carrier protein that facilitates the uptake of cholesterol from the blood.
These examples underscore the critical role of carrier proteins in maintaining cellular homeostasis and overall health.
Conclusion: Carriers are Essential for Life
Carrier proteins are essential for cellular function, enabling the transport of a wide variety of molecules across the plasma membrane. Through facilitated diffusion and active transport, they maintain the precise balance of ions and metabolites necessary for cell survival and function. Understanding the mechanisms and regulation of carrier-mediated transport is crucial for comprehending fundamental cellular processes and developing treatments for diseases associated with transport defects. Further research continues to unravel the intricate details of these vital membrane proteins and their contributions to the complex workings of life.
Latest Posts
Latest Posts
-
How Many Neutrons Are In An Atom Of Carbon 14
Apr 17, 2025
-
The Alleles Of A Particular Gene Are Different
Apr 17, 2025
-
What Is The Correct Order Of Cellular Respiration
Apr 17, 2025
-
Where Is Starch Stored In The Plant
Apr 17, 2025
-
What Is The Purest Form Of Matter
Apr 17, 2025
Related Post
Thank you for visiting our website which covers about Carriers Transport Solutes Across The Plasma Membrane By . We hope the information provided has been useful to you. Feel free to contact us if you have any questions or need further assistance. See you next time and don't miss to bookmark.