Branch Of Biology That Studies Heredity
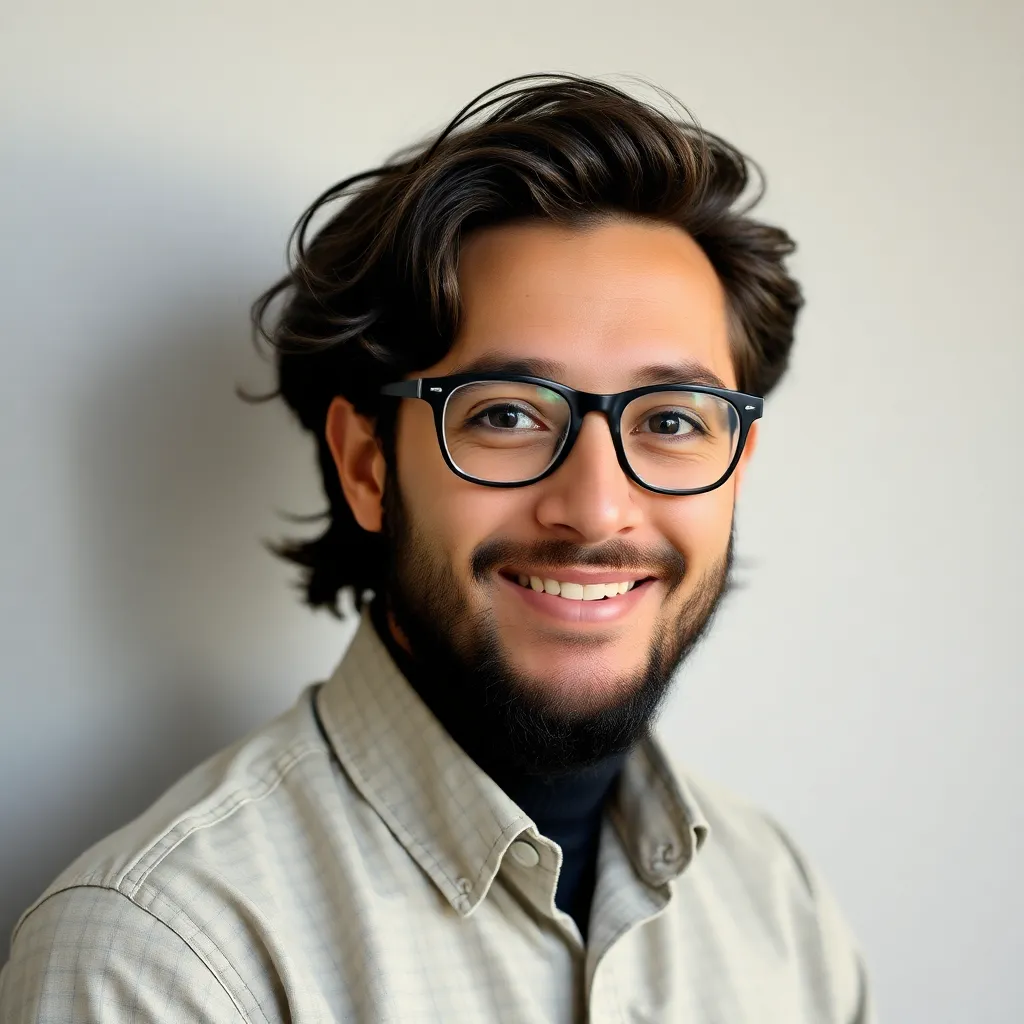
News Leon
Mar 14, 2025 · 7 min read

Table of Contents
Genetics: Unraveling the Secrets of Heredity
Genetics, a cornerstone of modern biology, is the branch of biology that studies heredity, the process by which characteristics are passed from one generation to the next. This fascinating field delves into the intricate mechanisms that govern the transmission of traits, from physical attributes like eye color and height to complex characteristics like susceptibility to diseases. Understanding genetics is crucial not only for comprehending the diversity of life but also for advancing medicine, agriculture, and many other fields.
The Fundamental Unit: Genes and DNA
At the heart of genetics lies the gene, the fundamental unit of heredity. Genes are specific sequences of deoxyribonucleic acid (DNA), a molecule that holds the blueprint for life. DNA is a double-helix structure composed of four nucleotide bases: adenine (A), guanine (G), cytosine (C), and thymine (T). The specific order, or sequence, of these bases along a DNA molecule determines the genetic code.
The Structure of DNA: A Closer Look
The double helix structure of DNA is crucial to its function. The two strands are held together by hydrogen bonds between complementary base pairs: A always pairs with T, and G always pairs with C. This complementary base pairing allows DNA to replicate itself accurately during cell division, ensuring that genetic information is faithfully passed on to daughter cells.
Genes and Proteins: The Central Dogma of Molecular Biology
Genes don't directly determine traits; instead, they provide instructions for building proteins. Proteins are the workhorses of the cell, carrying out a vast array of functions, from catalyzing biochemical reactions to providing structural support. The process of translating genetic information into proteins is called gene expression, and it involves two main steps:
- Transcription: The DNA sequence of a gene is copied into a messenger RNA (mRNA) molecule.
- Translation: The mRNA molecule is used as a template to assemble a chain of amino acids, forming a protein.
The specific sequence of amino acids in a protein determines its three-dimensional structure and, consequently, its function. Variations in the DNA sequence of a gene can lead to changes in the amino acid sequence of the protein, potentially altering its function and resulting in different traits.
Mendelian Genetics: The Foundation of Heredity
Gregor Mendel, a 19th-century monk, is considered the "father of genetics" for his groundbreaking experiments with pea plants. Mendel's work laid the foundation for understanding the basic principles of heredity, which are now known as Mendelian genetics.
Mendel's Laws: Segregation and Independent Assortment
Mendel's experiments revealed two fundamental laws of inheritance:
- The Law of Segregation: Each gene has two alleles (alternative forms of a gene), one inherited from each parent. These alleles segregate (separate) during gamete (sperm and egg) formation, so each gamete receives only one allele for each gene.
- The Law of Independent Assortment: Alleles for different genes segregate independently of each other during gamete formation. This means that the inheritance of one trait does not influence the inheritance of another trait.
Genotype and Phenotype: Understanding Genetic Expression
Mendel's work also distinguished between genotype and phenotype.
- Genotype: The genetic makeup of an organism, represented by the combination of alleles it possesses for a particular gene.
- Phenotype: The observable characteristics of an organism, determined by its genotype and the environment.
For example, a pea plant might have a genotype of "TT" (homozygous dominant) or "Tt" (heterozygous) for the gene controlling flower color, both resulting in a purple phenotype (purple flowers). Only a "tt" (homozygous recessive) genotype would result in a white phenotype (white flowers).
Beyond Mendelian Genetics: Exploring Complex Inheritance Patterns
While Mendel's laws provide a solid foundation for understanding heredity, many traits are not determined by simple dominant and recessive alleles. Non-Mendelian inheritance patterns include:
Incomplete Dominance: A Blend of Traits
In incomplete dominance, heterozygotes exhibit an intermediate phenotype between the two homozygous phenotypes. For example, if a red flower (RR) is crossed with a white flower (WW), the offspring might have pink flowers (RW).
Codominance: Both Alleles Expressed
In codominance, both alleles are fully expressed in the heterozygote. A classic example is the AB blood type, where both A and B antigens are present on the red blood cells.
Multiple Alleles: More Than Two Versions of a Gene
Many genes have more than two alleles, resulting in multiple alleles. A prime example is the ABO blood group system, which is determined by three alleles: IA, IB, and i.
Pleiotropy: One Gene, Multiple Effects
Pleiotropy occurs when a single gene affects multiple traits. For example, a gene that affects pigmentation might also influence susceptibility to certain diseases.
Polygenic Inheritance: Traits Influenced by Many Genes
Polygenic inheritance involves traits controlled by multiple genes, each contributing a small effect. Height, skin color, and weight are examples of polygenic traits, showing continuous variation.
Epigenetics: Modifying Gene Expression Without Altering DNA Sequence
Epigenetics studies heritable changes in gene expression that do not involve alterations to the underlying DNA sequence. These changes can be influenced by environmental factors and can be passed on to subsequent generations.
Modern Genetic Techniques: Unraveling the Mysteries of Life
Advances in technology have revolutionized the field of genetics, providing powerful tools for studying heredity and its impact on various aspects of life.
DNA Sequencing: Reading the Genetic Code
DNA sequencing is a technique used to determine the precise order of nucleotides in a DNA molecule. This technology has enabled scientists to decipher the entire genomes of numerous organisms, including humans, paving the way for significant advancements in medicine and other fields.
Polymerase Chain Reaction (PCR): Amplifying DNA
PCR is a technique used to amplify specific DNA sequences, making it possible to study even tiny amounts of DNA. PCR is crucial for a wide range of applications, including forensic science, medical diagnostics, and genetic research.
Gene Cloning: Producing Multiple Copies of a Gene
Gene cloning involves creating multiple copies of a specific gene. This technology is essential for studying gene function, developing genetically modified organisms (GMOs), and producing therapeutic proteins.
CRISPR-Cas9: Gene Editing Technology
CRISPR-Cas9 is a revolutionary gene-editing technology that allows scientists to precisely modify DNA sequences. This powerful tool holds immense promise for treating genetic diseases and developing new therapies.
Applications of Genetics: Transforming Various Fields
The understanding of genetics has far-reaching implications for numerous fields:
Medicine: Diagnosing and Treating Genetic Diseases
Genetics plays a crucial role in diagnosing and treating genetic diseases. Genetic testing can identify individuals at risk for inherited conditions, allowing for early intervention and preventative measures. Gene therapy aims to correct genetic defects by introducing functional genes into cells.
Agriculture: Developing Improved Crops and Livestock
Genetics is used to develop improved crops and livestock with desirable traits, such as increased yield, disease resistance, and nutritional value. Genetic engineering techniques are employed to modify the genomes of plants and animals, leading to significant advancements in agriculture.
Forensic Science: Solving Crimes and Identifying Individuals
DNA fingerprinting, a technique based on variations in DNA sequences, is a powerful tool in forensic science. DNA evidence can be used to solve crimes, identify missing persons, and establish paternity.
The Future of Genetics: Exploring Uncharted Territories
Genetics is a rapidly evolving field, with ongoing research pushing the boundaries of our understanding. Future advancements are expected to lead to further breakthroughs in:
- Personalized medicine: Tailoring medical treatments to an individual's unique genetic makeup.
- Gene therapy: Developing more effective and safer gene therapies for a wider range of genetic diseases.
- Synthetic biology: Designing and engineering new biological systems with specific functions.
- Understanding complex traits: Delving into the genetic basis of complex traits like intelligence, behavior, and susceptibility to common diseases.
In conclusion, genetics is a pivotal field that has revolutionized our understanding of heredity and its impact on life. From Mendel's foundational experiments to the advanced techniques of modern genetics, the study of heredity continues to unravel the mysteries of life and drive progress in medicine, agriculture, and numerous other fields. The future of genetics holds immense promise for further advancements that will shape our world in profound ways.
Latest Posts
Related Post
Thank you for visiting our website which covers about Branch Of Biology That Studies Heredity . We hope the information provided has been useful to you. Feel free to contact us if you have any questions or need further assistance. See you next time and don't miss to bookmark.