An Electron That Collides With An Atom Will
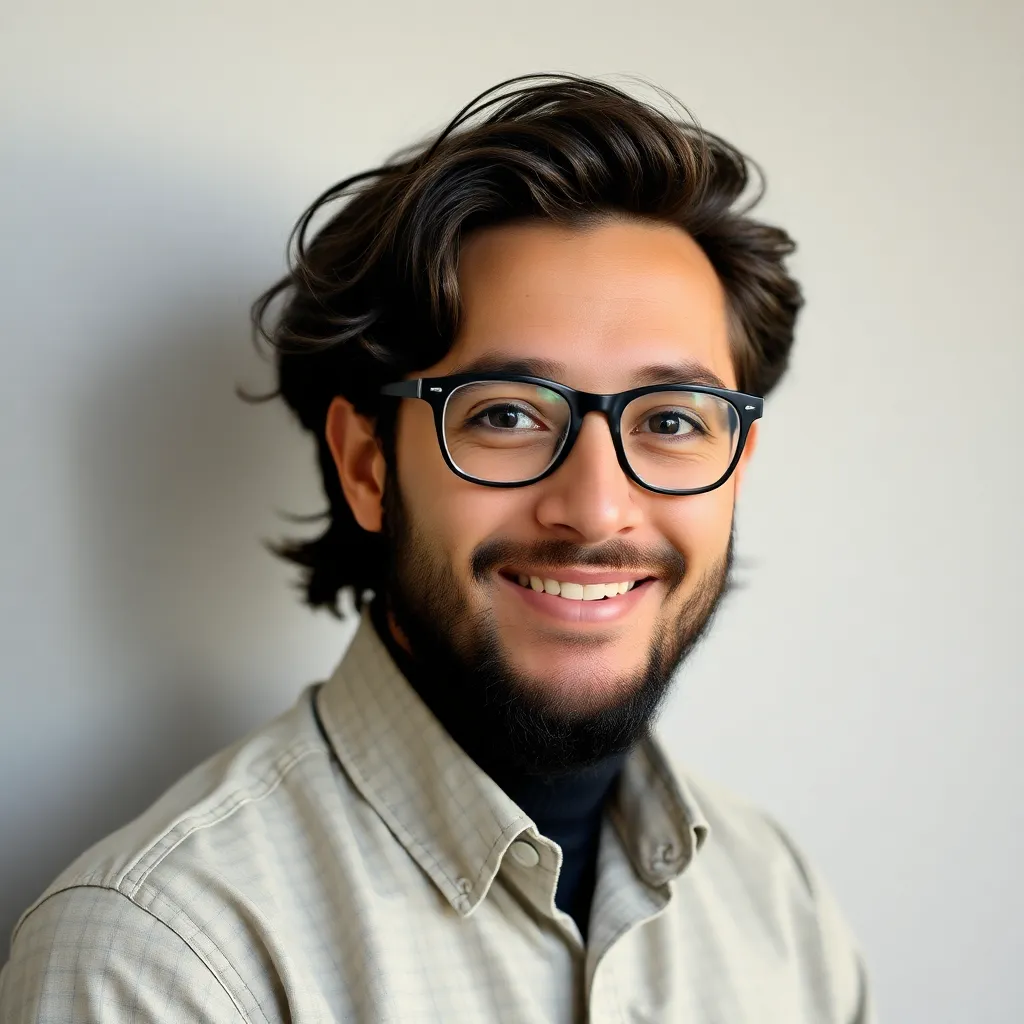
News Leon
Apr 17, 2025 · 7 min read

Table of Contents
When an Electron Collides with an Atom: A Deep Dive into Atomic Interactions
The seemingly simple scenario of an electron colliding with an atom opens a Pandora's Box of complex physical phenomena. Understanding this interaction is crucial to comprehending a vast range of scientific fields, from materials science and astrophysics to the development of advanced technologies like lasers and semiconductor devices. This article delves into the various outcomes of such collisions, exploring the underlying physics, the different types of interactions, and the significant consequences they have on the atomic and macroscopic levels.
The Diverse Outcomes of Electron-Atom Collisions
The fate of an electron after colliding with an atom is highly dependent on several factors: the electron's kinetic energy, the type of atom it interacts with, and even the atom's initial state (e.g., ground state or excited state). The collision can result in a variety of outcomes, broadly categorized as:
1. Elastic Scattering: A Bounce Without Excitation
In an elastic collision, the electron's kinetic energy is largely conserved. The electron interacts with the atom's electric field, altering its trajectory, but the atom itself remains largely unaffected, staying in its initial energy state. Think of it like two billiard balls colliding: they exchange momentum, changing their directions, but without any internal change to the balls themselves. This type of interaction is dominant at low electron energies. The scattering angle depends on the impact parameter (the distance of closest approach) and the strength of the atomic potential. Quantum mechanics plays a crucial role here, as the electron's wave-like nature dictates the probabilities of different scattering angles.
2. Inelastic Scattering: Energy Transfer and Excitation
Inelastic collisions are far more interesting and complex. Here, a significant portion of the electron's kinetic energy is transferred to the atom, causing it to transition to a higher energy state, often an excited state. The electron loses energy in this process, emerging from the collision with reduced kinetic energy. The energy difference between the initial and excited states of the atom exactly matches the energy lost by the electron. This process is highly specific; the electron must possess at least the minimum energy required to excite the atom to a particular energy level.
Excitation and Spectral Lines:
The excited atom is inherently unstable. It will quickly decay back to its ground state, emitting the excess energy as a photon of light. The energy of this photon directly corresponds to the energy difference between the excited and ground states. This is the fundamental principle behind atomic spectroscopy, where the characteristic spectral lines emitted by an element are used to identify it and determine its chemical composition. This is vital in astronomy, allowing us to analyze the composition of distant stars and galaxies. The intensity of these spectral lines can reveal information about the density and temperature of the emitting gas.
Ionization: Knocking Out an Electron
If the electron's kinetic energy exceeds the atom's ionization energy – the energy required to remove an electron from the atom – a different kind of inelastic scattering occurs: ionization. The collision results in the ejection of one or more electrons from the atom, creating a positively charged ion. The incident electron loses energy in this process, transferring enough energy to overcome the binding energy of the atomic electron. The ejected electron and the incident electron then both continue on their separate paths, carrying away kinetic energy.
Multiple Ionization:
Higher-energy collisions can lead to multiple ionization, whereby several electrons are ejected from the atom simultaneously. This process becomes increasingly probable at higher electron energies, generating highly charged ions. Understanding multiple ionization is crucial in fields such as plasma physics, where highly ionized plasmas are commonly encountered.
3. Bremsstrahlung Radiation: Deceleration and Photon Emission
When an electron passes close to the nucleus of an atom, it experiences a strong electrostatic attraction, leading to a significant deceleration. This deceleration causes the emission of electromagnetic radiation, a process known as bremsstrahlung radiation (German for "braking radiation"). The energy of the emitted photons is directly proportional to the change in the electron's kinetic energy. This is a continuous spectrum of radiation, unlike the discrete spectral lines generated by atomic excitation. Bremsstrahlung radiation is significant in high-energy processes, such as X-ray production in medical imaging and astronomical phenomena.
Factors Influencing Electron-Atom Collisions: A Deeper Look
Several factors significantly influence the probability and outcome of electron-atom collisions:
1. Electron Energy: The Key Determinant
The electron's kinetic energy is arguably the most crucial factor. Low-energy electrons predominantly undergo elastic scattering, while higher-energy electrons can cause excitation, ionization, or bremsstrahlung radiation. The energy thresholds for these different processes are specific to each atom and its electronic structure.
2. Atomic Structure: The Target's Influence
The atomic structure of the target atom greatly affects the collision outcome. The number of electrons, their orbital configurations, and the strength of the nuclear charge all influence the interaction. Atoms with loosely bound outer electrons are more susceptible to ionization at lower electron energies compared to atoms with tightly bound electrons.
3. Atomic State: Ground vs. Excited
The atom's initial state also plays a role. If the atom is already in an excited state, it requires less energy for further excitation or ionization. This can lead to different branching ratios of the various collision outcomes.
Applications and Significance: From Lasers to Astrophysics
Understanding electron-atom collisions is critical across numerous scientific and technological fields:
1. Laser Technology: Stimulated Emission
The principle of stimulated emission in lasers relies on electron-atom collisions to populate excited states of atoms. A high-energy electron beam or an electrical discharge can excite atoms to a higher energy level. When these excited atoms return to their ground state, they emit photons, and this process is amplified in a laser cavity, producing a coherent beam of light.
2. Plasma Physics: Ionization and Energy Transfer
Electron-atom collisions are fundamental to understanding plasma behavior. In plasmas, atoms are ionized by high-energy electrons, leading to the formation of ions and free electrons. The energy transfer processes during these collisions affect the temperature and properties of the plasma. This is crucial in fusion research and plasma-based technologies.
3. Astrophysics: Stellar Spectra and Element Abundance
Electron-atom collisions are essential in astrophysical studies to analyze stellar spectra and determine the abundance of elements in stars and nebulae. The spectral lines emitted by atoms as they undergo excitation and de-excitation provide crucial information about the composition and physical conditions of celestial objects.
4. Materials Science: Radiation Damage and Material Properties
In materials science, electron-atom collisions are crucial in understanding radiation damage in materials. High-energy electrons can displace atoms in the material lattice, leading to defects and changes in the material's properties. This has implications for the design of radiation-resistant materials for applications in nuclear reactors and space exploration.
5. Semiconductor Technology: Carrier Generation
In semiconductor devices, electron-atom collisions contribute to the generation of electron-hole pairs. These pairs are responsible for the conduction of electricity in semiconductors and are essential to the functioning of many electronic devices.
Conclusion: A Fundamental Interaction with Far-Reaching Consequences
The seemingly simple interaction between an electron and an atom unfolds into a surprisingly rich tapestry of physical phenomena. From elastic scattering to ionization and bremsstrahlung radiation, these collisions play a fundamental role in shaping the world around us. Understanding the intricacies of these interactions is crucial for advancing our knowledge in diverse fields, leading to innovations in technology and a deeper understanding of the universe's workings. Further research continues to refine our models and provide a more complete picture of these fundamental atomic processes, ensuring ongoing progress in scientific and technological advancements.
Latest Posts
Latest Posts
-
Pepsinogen An Inactive Digestive Enzyme Is Secreted By The
Apr 19, 2025
-
Coordinate Values In This Quadrant Are X0 And Y0
Apr 19, 2025
-
One Mole Of Oxygen Gas At Stp Occupies 22 4
Apr 19, 2025
-
Choose The Right Phrase To Complete The Sentence
Apr 19, 2025
-
Is Thymine A Purine Or Pyrimidine
Apr 19, 2025
Related Post
Thank you for visiting our website which covers about An Electron That Collides With An Atom Will . We hope the information provided has been useful to you. Feel free to contact us if you have any questions or need further assistance. See you next time and don't miss to bookmark.