A Voltaic Cell Converts Chemical Energy To
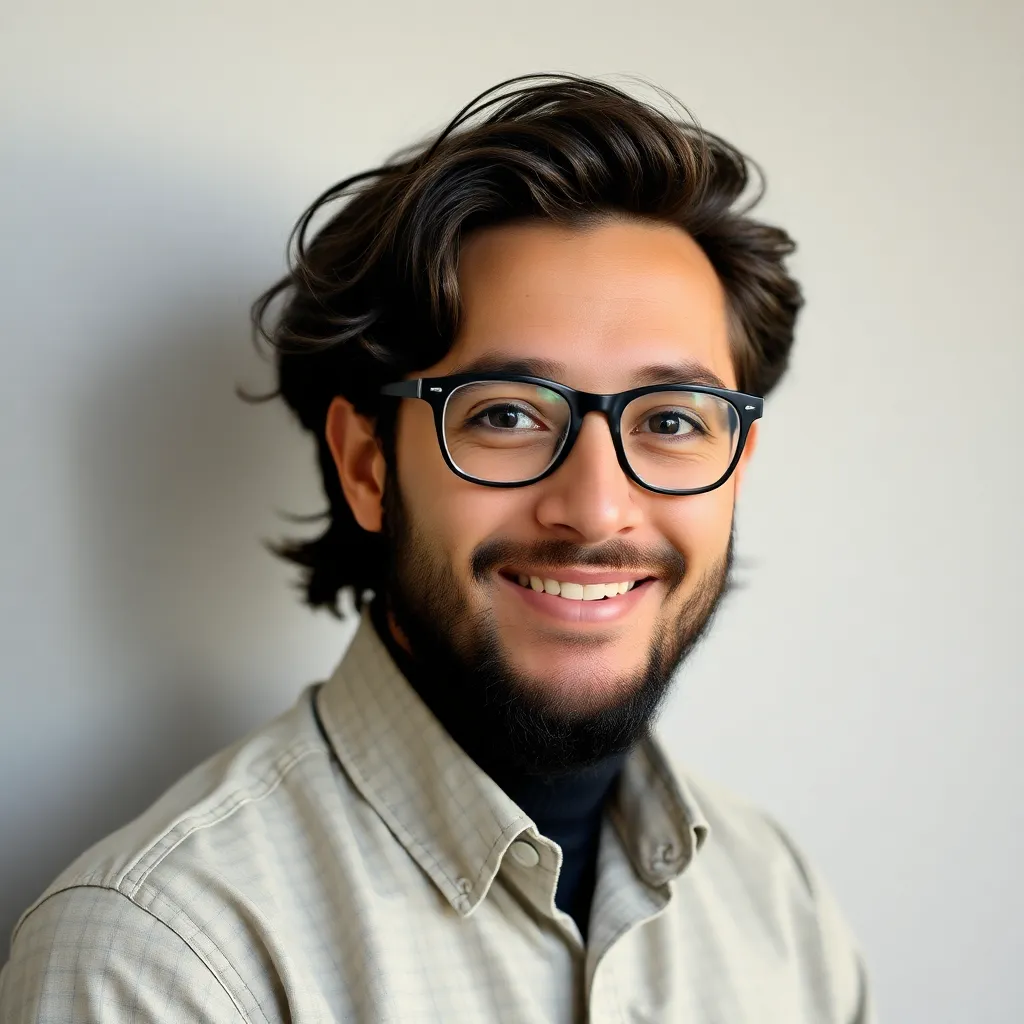
News Leon
Apr 06, 2025 · 7 min read
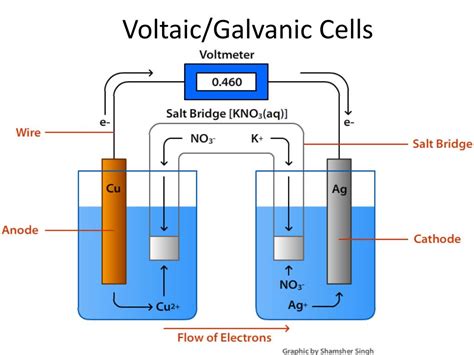
Table of Contents
A Voltaic Cell Converts Chemical Energy to Electrical Energy: A Deep Dive
A voltaic cell, also known as a galvanic cell, is an electrochemical device that converts chemical energy into electrical energy. This fascinating process underpins numerous applications in our daily lives, from powering our electronic devices to enabling essential industrial processes. Understanding the fundamental principles behind voltaic cells is crucial for appreciating their widespread impact. This comprehensive article will delve deep into the intricacies of voltaic cells, exploring their construction, operation, applications, and limitations.
Understanding the Core Principles: Oxidation and Reduction
At the heart of a voltaic cell lies a redox reaction – a chemical reaction involving the transfer of electrons. This transfer doesn't happen directly between reactants; instead, it's mediated through an external circuit, generating a flow of electrons that constitutes the electrical current. The reaction involves two key processes:
Oxidation: The Loss of Electrons
Oxidation is the process where a substance loses electrons. The substance undergoing oxidation is called the reducing agent because it reduces another substance by providing electrons. It experiences an increase in oxidation state (a measure of the apparent charge of an atom in a molecule).
Reduction: The Gain of Electrons
Reduction is the process where a substance gains electrons. The substance undergoing reduction is called the oxidizing agent because it oxidizes another substance by accepting its electrons. It experiences a decrease in oxidation state.
Remember: Oxidation and reduction always occur simultaneously; one cannot happen without the other. This coupled process is termed a redox reaction.
The Anatomy of a Voltaic Cell
A typical voltaic cell comprises several key components:
1. Electrodes: The Sites of Redox Reactions
Electrodes are conductive materials that facilitate the electron transfer. There are two electrodes:
- Anode: The electrode where oxidation occurs. Electrons are released at the anode.
- Cathode: The electrode where reduction occurs. Electrons are consumed at the cathode.
2. Electrolytes: The Ion Conductors
Electrolytes are solutions (or sometimes molten salts) containing ions that conduct electricity. They provide a pathway for the movement of ions to balance the charge changes occurring at the electrodes. Each electrode typically sits immersed in its own electrolyte solution, which may be the same or different depending on the specific cell design.
3. Salt Bridge (or Porous Membrane): Maintaining Electrical Neutrality
The salt bridge (or a porous membrane) connects the two half-cells, preventing direct mixing of the electrolytes while allowing the flow of ions. This maintains electrical neutrality within each half-cell. Without the salt bridge, the build-up of charge at the electrodes would quickly halt the electron flow.
4. External Circuit: The Pathway for Electron Flow
The external circuit connects the two electrodes, providing a pathway for the electrons to flow from the anode (where they are released during oxidation) to the cathode (where they are consumed during reduction). This electron flow constitutes the electric current that powers external devices.
How a Voltaic Cell Works: A Step-by-Step Explanation
Let's consider a classic example: the Daniell cell, a simple voltaic cell consisting of a zinc electrode in a zinc sulfate solution and a copper electrode in a copper sulfate solution, connected by a salt bridge.
-
Oxidation at the Anode: At the zinc electrode (anode), zinc atoms lose two electrons to form zinc ions (Zn²⁺), which dissolve into the zinc sulfate solution. This is represented by the half-reaction:
Zn(s) → Zn²⁺(aq) + 2e⁻
-
Electron Flow: The electrons released at the anode travel through the external circuit to the copper electrode (cathode).
-
Reduction at the Cathode: At the copper electrode (cathode), copper ions (Cu²⁺) from the copper sulfate solution gain two electrons to form copper atoms, which deposit onto the copper electrode. This is represented by the half-reaction:
Cu²⁺(aq) + 2e⁻ → Cu(s)
-
Ion Migration through the Salt Bridge: To maintain electrical neutrality, ions migrate through the salt bridge. Anions (negatively charged ions) move towards the anode to balance the positive charge from the zinc ions, while cations (positively charged ions) move towards the cathode to balance the consumption of copper ions.
-
Overall Cell Reaction: Combining the two half-reactions gives the overall cell reaction:
Zn(s) + Cu²⁺(aq) → Zn²⁺(aq) + Cu(s)
This overall reaction shows the spontaneous transfer of electrons from zinc to copper ions, resulting in the generation of electrical energy.
Cell Potential and Standard Reduction Potentials
The driving force behind the electron flow in a voltaic cell is the difference in the tendency of the two electrodes to gain or lose electrons. This is quantified by the cell potential (Ecell), which is measured in volts (V). The cell potential is the difference between the reduction potentials of the two half-reactions:
Ecell = E°cathode - E°anode
where E°cathode and E°anode are the standard reduction potentials of the cathode and anode half-reactions, respectively. Standard reduction potentials are tabulated values measured under standard conditions (25°C, 1 atm pressure, 1 M concentration of ions). A positive Ecell indicates a spontaneous reaction (a voltaic cell), while a negative Ecell indicates a non-spontaneous reaction (requiring external energy).
Factors Affecting Cell Potential
Several factors can influence the cell potential of a voltaic cell:
-
Concentration of Electrolytes: Changes in the concentration of ions in the electrolyte solutions will affect the cell potential. The Nernst equation describes this relationship quantitatively.
-
Temperature: Temperature also affects the cell potential, usually increasing it with increasing temperature.
-
Electrode Material: The choice of electrode materials significantly influences the cell potential, as it determines the reduction potentials of the half-reactions.
-
Pressure (for gas electrodes): If a gas is involved in a half-reaction, the partial pressure of the gas will influence the cell potential.
Types of Voltaic Cells
Many different types of voltaic cells exist, each designed for specific applications. Some common examples include:
-
Primary Cells: These are disposable cells that cannot be recharged. Common examples include alkaline batteries and zinc-carbon batteries.
-
Secondary Cells: These are rechargeable cells that can be recharged by reversing the chemical reaction through the application of an external electric current. Examples include lead-acid batteries and lithium-ion batteries.
-
Fuel Cells: These cells generate electricity by continuously supplying reactants (fuel and oxidant) to the electrodes. Hydrogen fuel cells are a prominent example.
Applications of Voltaic Cells
Voltaic cells have a vast array of applications in modern society, including:
-
Portable Electronic Devices: Powering laptops, smartphones, and other portable devices.
-
Automotive Industry: Powering electric vehicles and hybrid vehicles.
-
Grid-Scale Energy Storage: Storing renewable energy (solar, wind) for later use.
-
Medical Devices: Powering pacemakers, hearing aids, and other implantable devices.
-
Industrial Processes: Electroplating, electrorefining, and other electrochemical processes.
-
Space Exploration: Powering spacecraft and satellites.
Limitations of Voltaic Cells
Despite their many advantages, voltaic cells have some limitations:
-
Finite Lifespan: Primary cells have a limited lifespan, as the reactants are eventually consumed.
-
Environmental Concerns: Some battery types contain hazardous materials that pose environmental challenges upon disposal.
-
Energy Density: The amount of energy that can be stored per unit volume or mass is limited for some battery types.
-
Recharging Limitations: Rechargeable batteries can degrade over time with repeated charging cycles.
-
Safety Concerns: Improper handling or malfunction can lead to safety hazards, such as overheating or leakage.
The Future of Voltaic Cells
Research and development efforts continue to improve the performance and sustainability of voltaic cells. Areas of focus include:
-
Improved Energy Density: Developing batteries with higher energy density to extend the operating time of devices.
-
Faster Charging Times: Reducing the time required to recharge rechargeable batteries.
-
Enhanced Safety: Designing safer batteries to minimize the risk of fires or explosions.
-
Sustainable Materials: Utilizing environmentally friendly materials in battery manufacturing and developing methods for safe recycling.
-
Solid-State Batteries: Exploring solid-state electrolytes to enhance safety and improve performance.
Conclusion: A Powerful Technology with Ongoing Evolution
Voltaic cells are a cornerstone of modern technology, enabling the widespread use of portable electronic devices, electric vehicles, and numerous other applications. While existing technologies face limitations, ongoing research and development continue to push the boundaries of battery technology, promising even more efficient, safer, and sustainable energy storage solutions in the future. The fundamental principles of oxidation-reduction reactions underpinning their operation remain central to ongoing advancements in this vital field. Understanding these principles is essential for appreciating the impact of voltaic cells and the exciting possibilities that lie ahead in the realm of electrochemical energy conversion.
Latest Posts
Related Post
Thank you for visiting our website which covers about A Voltaic Cell Converts Chemical Energy To . We hope the information provided has been useful to you. Feel free to contact us if you have any questions or need further assistance. See you next time and don't miss to bookmark.