A Tiny Gap That Separates Two Neurons
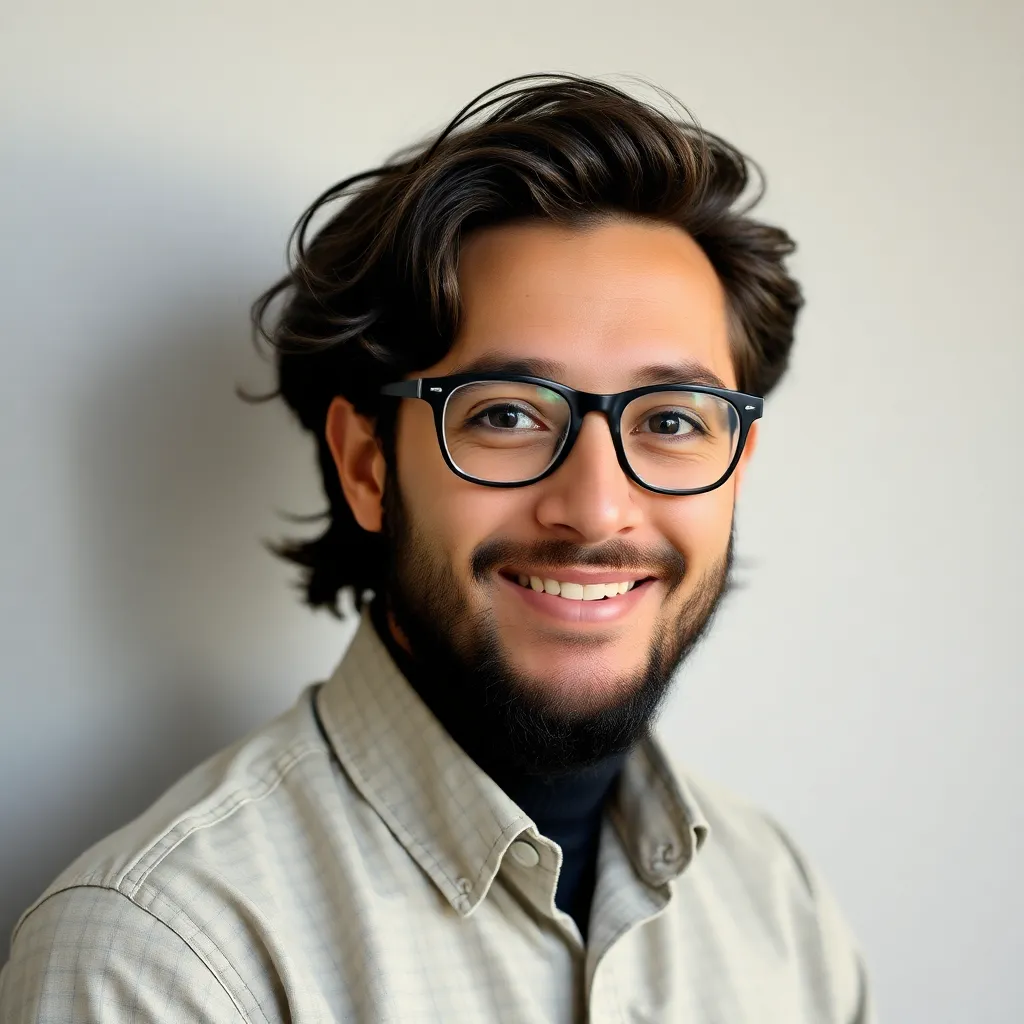
News Leon
Apr 04, 2025 · 7 min read
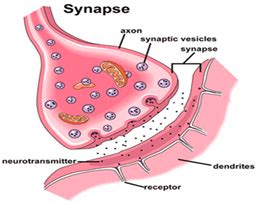
Table of Contents
The Tiny Gap: Exploring the Synapse and its Crucial Role in Neural Communication
The human brain, a marvel of biological engineering, houses approximately 86 billion neurons. These specialized cells, the fundamental units of the nervous system, don't actually touch each other. Instead, they communicate across a minuscule gap, a space so small it's almost imperceptible yet profoundly significant: the synapse. This seemingly insignificant chasm is the site of intricate molecular interactions, the very foundation of learning, memory, and virtually all aspects of our cognitive function. Understanding the synapse, this tiny gap that separates two neurons, is crucial to understanding the complexities of the brain itself.
Delving into the Synaptic Cleft: Structure and Function
The synapse isn't just empty space; it's a highly structured region with distinct components vital for communication. The two neurons involved are the presynaptic neuron, which transmits the signal, and the postsynaptic neuron, which receives it. Separating them is the synaptic cleft, a fluid-filled space approximately 20-40 nanometers wide—a distance smaller than the wavelength of visible light. This incredibly narrow gap ensures efficient signal transmission while allowing for sophisticated control mechanisms.
The Presynaptic Terminal: The Message Sender
The presynaptic neuron's terminal houses synaptic vesicles, tiny membrane-bound sacs brimming with neurotransmitters. These chemical messengers are the key players in neuronal communication. The arrival of an electrical signal, known as an action potential, at the presynaptic terminal triggers a cascade of events leading to the release of neurotransmitters into the synaptic cleft. This process is meticulously regulated, ensuring precise control over the signal strength and timing.
The Synaptic Cleft: The Communication Bridge
The synaptic cleft itself isn't passive; it's a dynamic environment containing various enzymes and other molecules influencing neurotransmitter diffusion, breakdown, and reuptake. The precise concentration and duration of neurotransmitters in this tiny gap are critical, dictating the strength and duration of the signal transmitted to the postsynaptic neuron. The size and composition of the cleft can even vary depending on the type of synapse and its activity level.
The Postsynaptic Receptor: The Message Receiver
On the postsynaptic neuron's membrane, specialized receptors await the neurotransmitters. These receptors are protein molecules that bind specifically to particular neurotransmitters, like a lock and key. Binding of the neurotransmitter initiates a change in the postsynaptic neuron's membrane potential, potentially triggering a new action potential and propagating the signal onward. Different types of receptors produce varying effects, allowing for complex integration of signals.
Types of Synapses: Diversity in Communication
Synapses aren't all created equal; they exhibit remarkable diversity in their structure and function. Two main types exist: chemical synapses and electrical synapses.
Chemical Synapses: The Dominant Players
Chemical synapses, the most prevalent type, rely on the release of neurotransmitters to transmit signals across the synaptic cleft. This mechanism allows for signal amplification, integration, and modulation, making it ideally suited for complex information processing. The chemical nature of the communication introduces a delay, but this delay is crucial for complex processing of information.
Electrical Synapses: Fast and Direct Communication
Electrical synapses, on the other hand, utilize direct electrical coupling between neurons through specialized protein channels called gap junctions. These junctions allow ions to flow directly from one neuron to the next, enabling extremely rapid signal transmission. While faster, electrical synapses lack the flexibility and regulatory mechanisms of chemical synapses, limiting their roles to situations requiring rapid, coordinated responses.
The Synapse in Action: A Symphony of Molecular Interactions
The process of neurotransmitter release and receptor binding is far from simple; it's a carefully orchestrated symphony of molecular interactions. Let's explore this process in detail:
- Action Potential Arrival: An action potential, a wave of electrical depolarization, reaches the presynaptic terminal.
- Calcium Influx: The depolarization triggers the opening of voltage-gated calcium channels, allowing calcium ions (Ca2+) to rush into the presynaptic terminal.
- Vesicle Fusion: The influx of Ca2+ triggers a series of molecular events culminating in the fusion of synaptic vesicles with the presynaptic membrane.
- Neurotransmitter Release: Neurotransmitters are released into the synaptic cleft through exocytosis.
- Diffusion Across the Cleft: Neurotransmitters diffuse across the tiny gap and bind to receptors on the postsynaptic membrane.
- Postsynaptic Potential: Receptor binding induces changes in the postsynaptic membrane potential, leading to either excitatory postsynaptic potentials (EPSPs) or inhibitory postsynaptic potentials (IPSPs).
- Signal Integration: The postsynaptic neuron integrates multiple EPSPs and IPSPs, determining whether it will fire an action potential.
- Neurotransmitter Removal: Neurotransmitters are removed from the synaptic cleft through various mechanisms, including reuptake by presynaptic transporters, enzymatic degradation, or diffusion away from the synapse.
The Synapse: A Dynamic Entity, Shaped by Experience
The synapse is not a static structure; its properties are constantly modified by experience. This plasticity, the ability of synapses to strengthen or weaken over time, is the neural basis of learning and memory.
Long-Term Potentiation (LTP): Strengthening the Connection
Long-term potentiation (LTP) is a persistent strengthening of synapses based on recent patterns of activity. It's a key mechanism for forming memories, allowing the brain to retain and recall information. This involves changes in the number and sensitivity of postsynaptic receptors, as well as structural changes in the synapse itself.
Long-Term Depression (LTD): Weakening the Connection
Long-term depression (LTD) is the counterpart of LTP, representing a long-lasting weakening of synapses. It's crucial for refining neural circuits and preventing the saturation of synaptic strength. LTD involves reducing the number or sensitivity of postsynaptic receptors, also resulting in structural modifications at the synapse.
Synaptic Dysfunction: Implications for Neurological and Psychiatric Disorders
Disruptions in synaptic function are implicated in a wide range of neurological and psychiatric disorders. These disorders highlight the critical role the synapse plays in maintaining healthy brain function.
Alzheimer's Disease: Impaired Synaptic Transmission
In Alzheimer's disease, the accumulation of amyloid plaques and neurofibrillary tangles disrupts synaptic transmission, leading to cognitive decline and memory loss. The resulting loss of synaptic connections underlies the progressive deterioration of cognitive abilities.
Parkinson's Disease: Dopamine Depletion
Parkinson's disease involves the degeneration of dopamine-producing neurons in the substantia nigra, leading to a deficiency in dopamine at synapses in the striatum. This dopamine depletion causes the motor symptoms characteristic of the disease.
Schizophrenia: Glutamate and Dopamine Imbalance
Schizophrenia is believed to involve disruptions in the balance of glutamate and dopamine neurotransmission at various synapses throughout the brain. This imbalance is thought to contribute to the positive, negative, and cognitive symptoms of the disorder.
Depression: Dysregulation of Neurotransmitter Systems
Depression is associated with dysregulation of multiple neurotransmitter systems, including serotonin, norepinephrine, and dopamine. These imbalances at synapses lead to mood disturbances, loss of motivation, and cognitive impairments.
The Future of Synaptic Research: Unveiling the Brain's Secrets
Research on the synapse continues to advance rapidly, revealing the intricate mechanisms that govern neural communication. New technologies allow scientists to probe the synapse with unprecedented detail, providing insights into its structure, function, and dysfunction. Understanding the synapse at the molecular level promises to revolutionize our understanding of brain function and provide novel therapeutic strategies for neurological and psychiatric disorders. Areas of active research include:
- Advanced imaging techniques: Techniques like super-resolution microscopy are allowing visualization of synaptic structures and dynamics with increasing detail.
- Optogenetics: This technique uses light to control the activity of specific neurons, providing precise control over synaptic transmission.
- Computational modeling: Computational models are being developed to simulate the complex interactions within synapses and predict their behavior under various conditions.
The tiny gap that separates two neurons, the synapse, is far from simple. It is a dynamic, multifaceted structure that plays a critical role in brain function, learning, and memory. By continuing to unravel its complexities, scientists are paving the way for innovative treatments for neurological and psychiatric disorders, offering hope for those affected by these devastating conditions. Further exploration into this remarkable structure promises to shed even more light on the intricate workings of the brain and the profound influence of this tiny gap on our thoughts, feelings, and actions. The journey of understanding the synapse is ongoing, and the discoveries to come are sure to reshape our understanding of the human mind.
Latest Posts
Latest Posts
-
Which Hormone Aids In Water Resorption
Apr 10, 2025
-
5000 Grams Equals How Many Kilograms
Apr 10, 2025
-
What Is The Fraction Of 150
Apr 10, 2025
-
Determine The Bond Order For The N2 Ion
Apr 10, 2025
-
Which Of The Following Issues Is Related To Microeconomics
Apr 10, 2025
Related Post
Thank you for visiting our website which covers about A Tiny Gap That Separates Two Neurons . We hope the information provided has been useful to you. Feel free to contact us if you have any questions or need further assistance. See you next time and don't miss to bookmark.