A Thread Like Structure Of Dna That Carries Genes
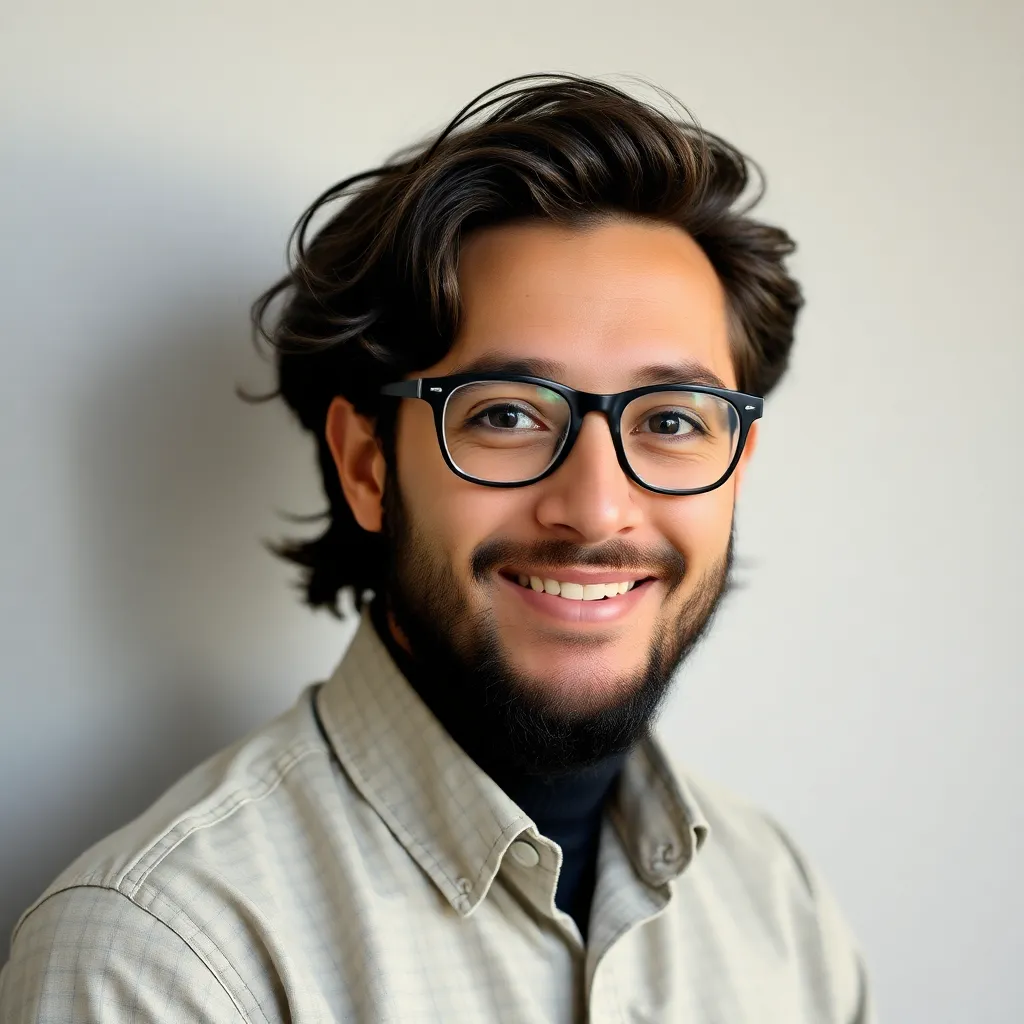
News Leon
Apr 12, 2025 · 6 min read

Table of Contents
Decoding the Double Helix: A Deep Dive into the Thread-Like Structure of DNA That Carries Genes
The very foundation of life, the blueprint for every organism, resides within a seemingly simple structure: deoxyribonucleic acid (DNA). This thread-like molecule, organized into chromosomes, carries the genetic instructions for the development, functioning, growth, and reproduction of all known organisms and many viruses. Understanding its structure is key to understanding life itself. This article delves into the intricate details of DNA's structure, its role in carrying genes, and the implications of its remarkable design.
The Double Helix: A Masterpiece of Molecular Architecture
DNA's iconic double helix structure, discovered by Watson and Crick in 1953, is far more than just a pretty picture. It's a meticulously crafted arrangement that dictates how genetic information is stored, accessed, and replicated. Let's break down its key components:
1. Nucleotides: The Building Blocks
DNA is a polymer, meaning it's composed of repeating subunits called nucleotides. Each nucleotide consists of three parts:
- A deoxyribose sugar: A five-carbon sugar molecule that forms the backbone of the DNA strand.
- A phosphate group: This negatively charged group links the sugar molecules together, creating the sugar-phosphate backbone.
- A nitrogenous base: This is the crucial part that carries genetic information. There are four types of nitrogenous bases in DNA:
- Adenine (A)
- Guanine (G)
- Cytosine (C)
- Thymine (T)
These bases pair specifically with each other: A always pairs with T, and G always pairs with C. This specific pairing is crucial for DNA replication and gene expression.
2. The Sugar-Phosphate Backbone: The Structural Framework
The sugar and phosphate groups alternate to form the two strands of the double helix. This backbone provides structural support and stability to the DNA molecule. The negatively charged phosphate groups contribute to the molecule's overall negative charge, which is important for its interactions with proteins and other molecules.
3. Base Pairing: The Language of Life
The specific pairing of bases (A with T, and G with C) is held together by hydrogen bonds. These are relatively weak bonds, allowing the two strands to separate easily during DNA replication and transcription. However, the collective strength of numerous hydrogen bonds ensures the stability of the double helix.
4. The Double Helix: A Twist of Fate
The two strands of DNA are not parallel; they run antiparallel to each other, meaning that one strand runs in the 5' to 3' direction, while the other runs in the 3' to 5' direction. This arrangement is essential for DNA replication and the proper functioning of DNA polymerase, the enzyme that synthesizes new DNA strands. The twisting of these two antiparallel strands creates the characteristic double helix shape.
Genes: The Functional Units of Heredity
Within this magnificent double helix lies the genetic code, organized into functional units called genes. Genes are specific sequences of nucleotides that carry the instructions for building and maintaining an organism. They essentially act as the recipes for producing proteins, the workhorses of the cell.
1. The Central Dogma of Molecular Biology
The flow of genetic information from DNA to protein is described by the central dogma of molecular biology:
DNA → RNA → Protein
This process involves two key steps:
- Transcription: The DNA sequence of a gene is copied into a messenger RNA (mRNA) molecule. This process takes place in the nucleus of eukaryotic cells.
- Translation: The mRNA molecule travels to the ribosomes, where the genetic code is translated into a sequence of amino acids, forming a protein. This process takes place in the cytoplasm.
2. Gene Structure: Promoters, Exons, and Introns
Eukaryotic genes are more complex than prokaryotic genes. They contain several key regions:
- Promoter: This region upstream of the gene acts as a binding site for RNA polymerase, the enzyme that initiates transcription.
- Exons: These are the coding sequences of the gene, which are translated into protein.
- Introns: These are non-coding sequences within the gene that are transcribed but then spliced out of the mRNA before translation. Introns play a role in gene regulation and can influence protein diversity through alternative splicing.
3. Gene Regulation: Controlling Gene Expression
Not all genes are expressed at all times. Gene expression is tightly regulated to ensure that the right proteins are produced at the right time and in the right amount. This regulation can occur at various levels, including:
- Transcriptional regulation: Controlling the rate of transcription initiation.
- Post-transcriptional regulation: Affecting mRNA processing, stability, and translation.
- Post-translational regulation: Modifying the protein after it's synthesized.
DNA Replication: Preserving the Genetic Code
The precise replication of DNA is critical for the inheritance of genetic information. This process involves the unwinding of the double helix, the separation of the two strands, and the synthesis of two new complementary strands using the original strands as templates. The enzyme DNA polymerase plays a central role in this process.
The accuracy of DNA replication is incredibly high, with very few errors occurring during the process. This high fidelity is essential for maintaining the integrity of the genome and preventing mutations.
Mutations: Changes in the Genetic Code
Despite the high fidelity of DNA replication, errors can occur, leading to mutations. These changes in the DNA sequence can have various consequences, ranging from no effect to severe genetic disorders. Mutations can be caused by various factors, including:
- Spontaneous errors: Random mistakes during DNA replication.
- Mutagenic agents: Environmental factors such as radiation or chemicals that damage DNA.
Mutations can be classified as:
- Point mutations: Changes in a single nucleotide.
- Insertions: Adding nucleotides to the sequence.
- Deletions: Removing nucleotides from the sequence.
Chromosomes: Organizing the Genetic Material
DNA is not simply a long, unwound molecule in the cell. It's highly organized and packaged into structures called chromosomes. Chromosomes are composed of DNA tightly wound around proteins called histones. This packaging allows the vast amount of genetic information to be efficiently stored within the cell's nucleus.
Humans have 23 pairs of chromosomes, one set inherited from each parent. These chromosomes contain thousands of genes, each playing a specific role in the development and functioning of the organism.
Epigenetics: Beyond the DNA Sequence
While the DNA sequence itself carries the fundamental genetic information, the expression of genes can also be influenced by epigenetic modifications. These are heritable changes in gene expression that do not involve alterations to the DNA sequence itself. Epigenetic modifications include:
- DNA methylation: The addition of a methyl group to a DNA base, often silencing gene expression.
- Histone modification: Changes to the proteins around which DNA is wound, affecting gene accessibility.
Epigenetic modifications play an important role in development, disease, and even transgenerational inheritance.
Conclusion: The Enduring Legacy of DNA's Thread-Like Structure
The thread-like structure of DNA, with its elegant double helix and precisely arranged nucleotides, is a testament to the power of biological design. Its ability to store, replicate, and express genetic information is the foundation of life itself. Further research continues to unravel the complexities of DNA's structure and function, leading to breakthroughs in medicine, agriculture, and our understanding of the natural world. From understanding genetic diseases to developing gene therapies, the knowledge of this thread-like structure continues to shape our future. The journey to fully understand this remarkable molecule is ongoing, but the impact of this understanding is already profound and continues to grow.
Latest Posts
Latest Posts
-
Animals Eliminate Nitrogenous Waste To By
Apr 18, 2025
-
Cell Type Not Found In Areolar Connective Tissue
Apr 18, 2025
-
What Equalizes Pressure On Both Sides Of The Tympanic Membrane
Apr 18, 2025
-
Which Of The Following Are Sources Of Cash
Apr 18, 2025
-
Acromial End Of Clavicle Articulates With
Apr 18, 2025
Related Post
Thank you for visiting our website which covers about A Thread Like Structure Of Dna That Carries Genes . We hope the information provided has been useful to you. Feel free to contact us if you have any questions or need further assistance. See you next time and don't miss to bookmark.