A Parallel Plate Capacitor Is Connected To A Battery
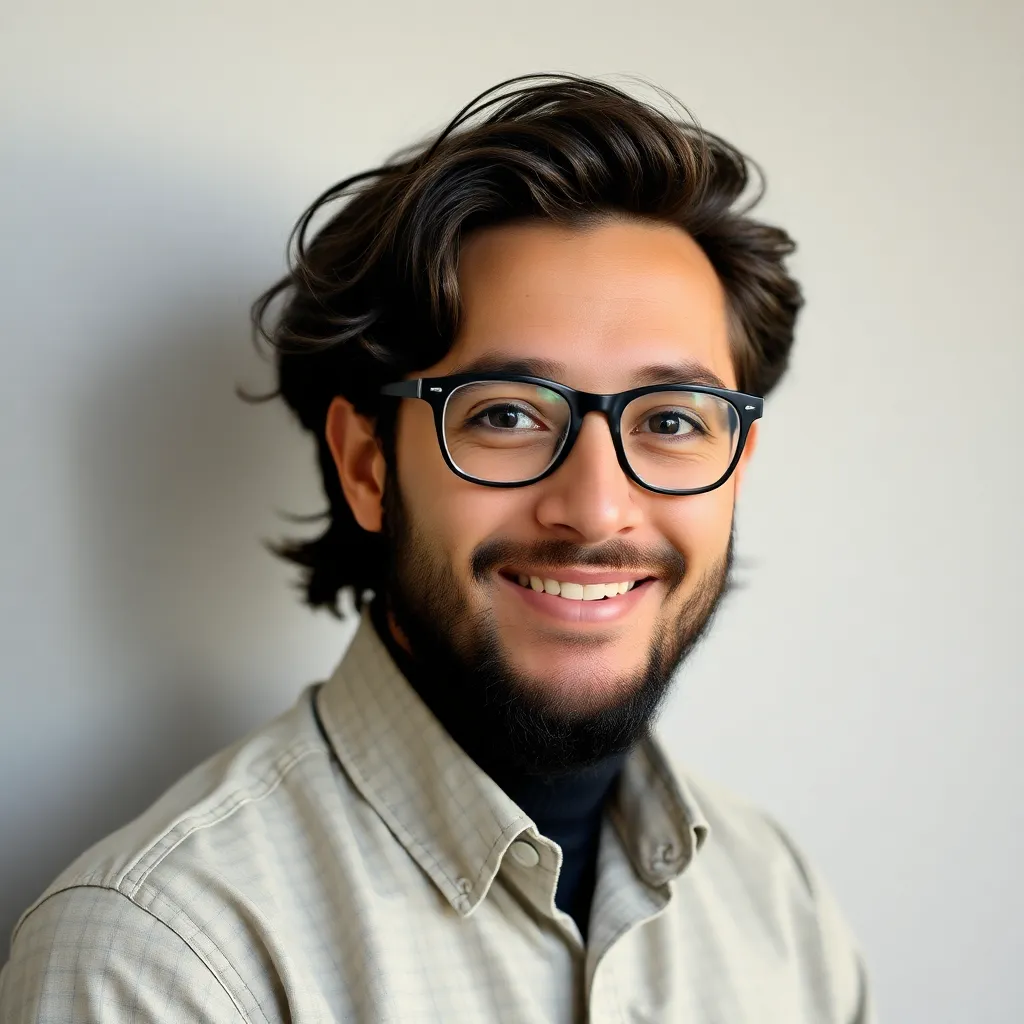
News Leon
Apr 16, 2025 · 6 min read

Table of Contents
A Parallel Plate Capacitor Connected to a Battery: A Deep Dive into Electrostatics
A parallel plate capacitor, a fundamental component in electronics and electromagnetism, exhibits fascinating behavior when connected to a battery. This seemingly simple setup offers a rich tapestry of concepts, from basic electrostatics to more advanced ideas about energy storage and dielectric materials. This article will delve into the intricacies of this system, exploring its behavior from a fundamental level to its practical applications.
Understanding the Basics: Capacitance and Charge Storage
At its core, a parallel plate capacitor consists of two conductive plates separated by an insulating material called a dielectric. When a battery is connected across the plates, a potential difference (voltage) is established between them. This voltage drives electrons from one plate (becoming positively charged) to the other (becoming negatively charged), resulting in a charge separation. The ability of a capacitor to store this charge is quantified by its capacitance (C), measured in Farads (F).
The Capacitance Formula:
The capacitance of a parallel plate capacitor is directly proportional to the area (A) of the plates and the permittivity (ε) of the dielectric material, and inversely proportional to the separation distance (d) between the plates:
C = εA/d
This formula highlights several crucial factors:
- Larger Plate Area (A): A larger plate area provides more surface area for charge accumulation, leading to higher capacitance.
- Smaller Plate Separation (d): Decreasing the distance between plates reduces the resistance to charge movement, thereby increasing capacitance.
- Higher Permittivity (ε): The permittivity of the dielectric material reflects its ability to store electrical energy. A higher permittivity material (like certain ceramics) allows for greater charge storage at a given voltage, leading to higher capacitance. Vacuum has the lowest permittivity, while materials like mica, ceramic, and Teflon have significantly higher values.
The Charging Process: A Dynamic Equilibrium
Connecting a capacitor to a battery initiates a dynamic process. Initially, a current flows readily, driven by the potential difference between the battery terminals and the uncharged capacitor plates. However, as charge accumulates on the plates, an opposing electric field builds up within the capacitor. This field counteracts the battery's voltage, gradually reducing the current flow.
The Time Constant (τ):
The charging process isn't instantaneous. It's governed by a time constant (τ), represented by the product of the resistance (R) in the circuit and the capacitance (C):
τ = RC
This time constant represents the time it takes for the capacitor voltage to reach approximately 63.2% of the battery voltage. After five time constants (5τ), the capacitor is considered fully charged, reaching approximately 99.3% of the battery voltage. This exponential charging behavior is described by:
V(t) = V₀(1 - e^(-t/τ))
Where:
- V(t) is the voltage across the capacitor at time t.
- V₀ is the battery voltage.
- e is the base of the natural logarithm.
Energy Storage: The Heart of Capacitor Functionality
One of the primary functions of a capacitor is its ability to store electrical energy. This energy is stored in the electric field between the capacitor plates. The energy (U) stored in a capacitor is given by:
U = ½CV²
This equation demonstrates that the energy stored is directly proportional to the capacitance and the square of the voltage across the capacitor. This means doubling the voltage quadruples the stored energy.
Dielectric Breakdown: A Limiting Factor
The dielectric material between the capacitor plates plays a crucial role in energy storage. However, every dielectric material has a breakdown voltage, the maximum voltage it can withstand before electrical breakdown occurs. Exceeding this voltage can lead to dielectric failure, short-circuiting the capacitor and potentially damaging the circuit. Choosing an appropriate dielectric material with a high breakdown voltage is essential for safe and reliable capacitor operation.
Discharging the Capacitor: Releasing Stored Energy
Once a capacitor is charged, it can be discharged by removing the battery and connecting the plates through a resistor or other load. The discharge process is also exponential, following a similar time constant (τ = RC) but with the voltage decaying according to:
V(t) = V₀e^(-t/τ)
This discharge process releases the stored energy, which can be used to power circuits, generate pulses, or perform other functions. The rate of discharge is determined by the resistance in the circuit; a lower resistance leads to faster discharge.
Practical Applications: From Simple Circuits to Complex Systems
Parallel plate capacitors find widespread applications across numerous fields:
- Filtering: Capacitors are crucial in filtering out unwanted frequencies in electronic circuits, smoothing out voltage fluctuations, and separating AC and DC signals.
- Energy Storage: They act as temporary energy reservoirs in power supplies, enabling circuits to operate during brief power interruptions.
- Timing Circuits: Capacitors, combined with resistors, form RC circuits that determine the timing of various electronic processes, vital for applications such as timers and oscillators.
- Coupling and Decoupling: Capacitors effectively couple signals between different stages in a circuit or decouple fluctuating voltages to prevent unwanted interference.
- Tuning Circuits: In radio receivers and other frequency-selective applications, variable capacitors are essential for tuning to specific frequencies.
- Power Factor Correction: In AC power systems, capacitors help improve the power factor, optimizing the efficiency of energy transfer.
Beyond the Ideal: Factors Affecting Real-World Performance
While the equations presented above provide a good understanding of the ideal parallel plate capacitor, several real-world factors can influence its performance:
- Fringing Effects: The electric field lines near the edges of the plates aren't perfectly uniform, leading to slight deviations from the idealized model.
- Non-uniform Dielectric: Imperfections and non-uniformities in the dielectric material can affect the capacitance and energy storage capacity.
- Temperature Dependence: The capacitance of a capacitor can vary with temperature changes due to alterations in the dielectric's permittivity and the dimensions of the plates.
- Parasitic Capacitance and Inductance: Real-world capacitors exhibit parasitic capacitance and inductance due to the wiring and components, affecting their high-frequency performance.
Exploring Variations: Different Capacitor Types
The basic parallel plate capacitor design has numerous variations, each tailored for specific applications:
- Ceramic Capacitors: These capacitors use ceramic materials as dielectrics, offering high capacitance in a small package.
- Film Capacitors: These utilize thin films of plastic or metal oxides as dielectrics, known for their stability and low losses.
- Electrolytic Capacitors: These have a higher capacitance than other types due to the use of an electrolytic solution as the dielectric, but they are polarized and must be connected with the correct polarity.
- Variable Capacitors: These allow for adjustable capacitance, making them useful in tuning circuits and other applications.
Conclusion: A Versatile and Essential Component
The parallel plate capacitor, despite its apparent simplicity, offers a wealth of fascinating electrostatic phenomena and serves as a fundamental component in numerous applications. Understanding its behavior, from charging and discharging dynamics to energy storage and practical applications, is crucial for anyone working with electronics or electromagnetism. This in-depth analysis highlights the importance of this ubiquitous component and its contributions to the modern technological landscape. Further research into the intricacies of dielectric materials and advanced capacitor designs will continue to refine and extend the applications of this vital electrical element. The journey from basic principles to real-world implementations underscores the beauty and practical significance of the parallel plate capacitor connected to a battery.
Latest Posts
Related Post
Thank you for visiting our website which covers about A Parallel Plate Capacitor Is Connected To A Battery . We hope the information provided has been useful to you. Feel free to contact us if you have any questions or need further assistance. See you next time and don't miss to bookmark.