A Battery Converts What Type Of Energy To Another
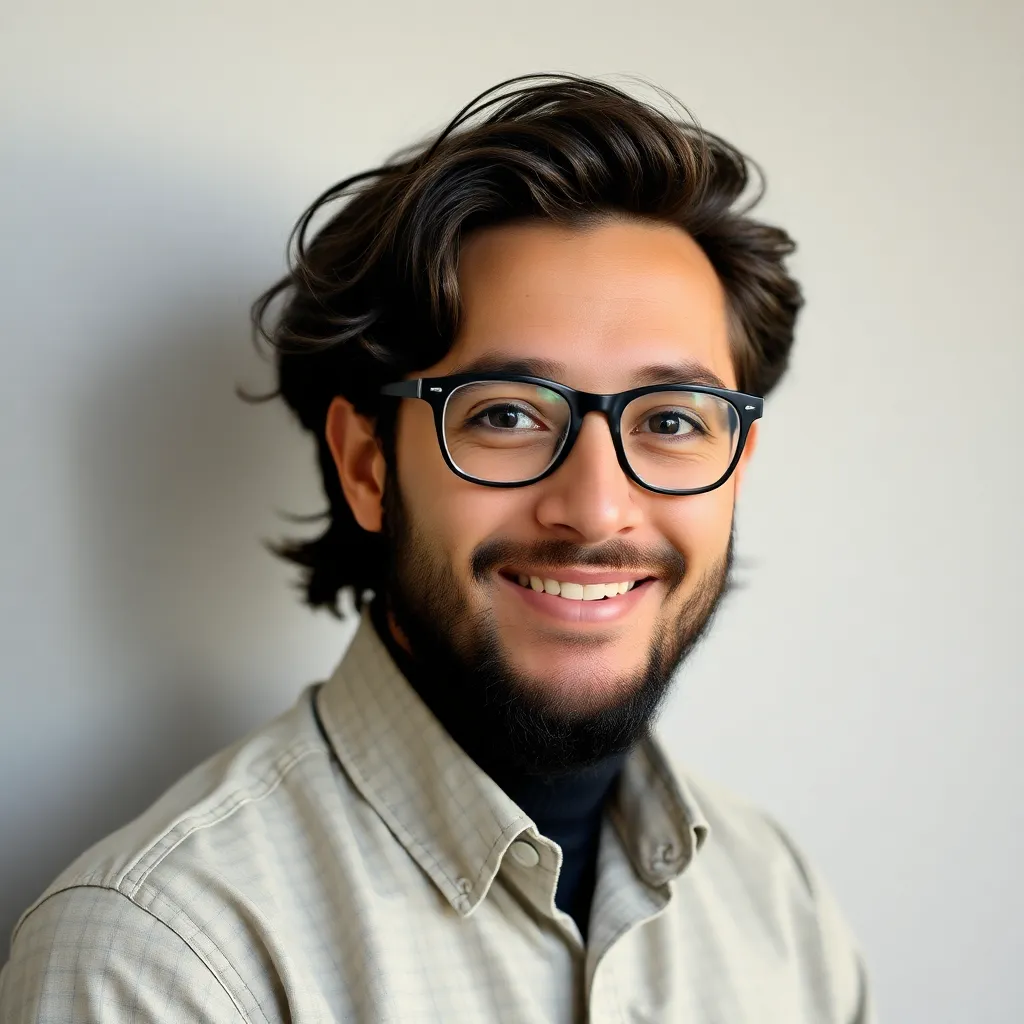
News Leon
Apr 22, 2025 · 6 min read

Table of Contents
A Battery Converts What Type of Energy to Another? The Complete Guide
Batteries are ubiquitous in modern life, powering everything from smartphones and laptops to electric vehicles and grid-scale energy storage. But how exactly do they work? At their core, batteries convert chemical energy into electrical energy. This seemingly simple process involves a complex interplay of chemical reactions and electrical potentials, making them fascinating devices worthy of deeper exploration. This article delves into the intricacies of this energy conversion, exploring the different types of batteries, their underlying chemical processes, and their role in the broader energy landscape.
Understanding the Basics: Chemical to Electrical Energy Conversion
The fundamental principle behind a battery’s operation is the electrochemical reaction. This involves a spontaneous redox reaction (reduction-oxidation reaction), where electrons are transferred between different chemical species. This electron transfer creates a flow of electric current, which is the electrical energy we harness.
A battery consists of several key components:
- Anode: The negative electrode, where oxidation (loss of electrons) occurs.
- Cathode: The positive electrode, where reduction (gain of electrons) occurs.
- Electrolyte: A conductive medium (liquid, paste, or solid) that allows the movement of ions between the anode and cathode, completing the electrical circuit.
- Separator: A porous membrane that prevents direct contact between the anode and cathode, while still allowing ion transport.
The chemical reaction within the battery is designed such that the electrons released at the anode are forced to travel through an external circuit (e.g., the circuit powering your phone) to reach the cathode. This movement of electrons constitutes the electrical current. The electrolyte facilitates the movement of ions to maintain electrical neutrality, balancing the charge transfer within the battery.
The Role of Redox Reactions
The heart of a battery's energy conversion lies in the redox reaction. Let's illustrate this with a simple example, the common zinc-carbon battery:
- Anode (Zinc): Zinc metal is oxidized, losing electrons: Zn → Zn²⁺ + 2e⁻
- Cathode (Carbon): Manganese dioxide (MnO₂) is reduced, gaining electrons: MnO₂ + H₂O + e⁻ → MnO(OH) + OH⁻
The electrons released from the oxidation of zinc at the anode travel through the external circuit to reach the cathode, where they reduce manganese dioxide. The movement of these electrons provides the electrical energy. The electrolyte (usually an ammonium chloride paste) allows the movement of ions (Zn²⁺ and OH⁻) to maintain charge balance.
Different Types of Batteries and Their Energy Conversion Mechanisms
Batteries come in various forms, each utilizing different chemical reactions and materials to achieve their energy conversion. Some common types include:
1. Primary Batteries (Single-Use):
These batteries are designed for single use and cannot be recharged. The chemical reactions within them are irreversible. Common examples include:
- Zinc-carbon batteries: These are inexpensive and widely used for low-power applications. As described above, their energy conversion relies on the redox reaction between zinc and manganese dioxide.
- Alkaline batteries: These offer higher energy density and longer lifespan than zinc-carbon batteries. They typically use zinc as the anode and manganese dioxide as the cathode, but with a different electrolyte (potassium hydroxide) leading to a more efficient reaction.
2. Secondary Batteries (Rechargeable):
Unlike primary batteries, secondary batteries can be recharged multiple times by reversing the electrochemical reactions. This is achieved by applying an external electrical current to the battery, forcing the electrons to flow in the opposite direction, regenerating the original chemical species. Popular examples include:
- Lead-acid batteries: These are widely used in automobiles, offering high current output and relatively low cost. The energy conversion involves the oxidation and reduction of lead and lead dioxide in a sulfuric acid electrolyte.
- Nickel-metal hydride (NiMH) batteries: These offer higher energy density than nickel-cadmium (NiCd) batteries and are environmentally friendlier. Their energy conversion involves the oxidation and reduction of nickel hydroxide and a metal hydride.
- Lithium-ion batteries (Li-ion): These are the dominant technology in portable electronics and electric vehicles, providing high energy density, long cycle life, and relatively low self-discharge. The energy conversion involves the intercalation and deintercalation of lithium ions between the anode and cathode materials, usually graphite and a metal oxide, respectively.
- Lithium-polymer batteries: A variation of lithium-ion batteries, using a polymer electrolyte instead of a liquid electrolyte. This offers improved safety and flexibility.
The Chemical Details: A Deeper Dive into Lithium-ion Batteries
Given their widespread use and technological importance, let's examine the chemical processes in lithium-ion batteries in more detail.
During discharge (energy release):
- Anode (Graphite): Lithium ions (Li⁺) are released from the graphite anode, leaving electrons behind. LiC₆ → 6C + Li⁺ + e⁻
- Electrolyte: Lithium ions migrate through the electrolyte to the cathode.
- Cathode (Metal Oxide, e.g., LiCoO₂): Lithium ions combine with the cathode material, accepting electrons from the external circuit. Li⁺ + e⁻ + LiCoO₂ → LiₓCoO₂ (x represents the number of lithium ions intercalated).
During charging (energy storage):
The process is reversed. An external electrical current forces electrons back to the anode, and lithium ions move back from the cathode to the anode, restoring the battery to its initial state.
Beyond Chemical Energy: Other Energy Conversion Aspects
While the primary focus is the conversion of chemical energy to electrical energy, other energy conversions are involved in the battery's operation:
- Heat Generation: Electrochemical reactions are not perfectly efficient. Some energy is lost as heat during both charging and discharging. This heat generation needs to be managed to prevent overheating and potential safety hazards.
- Internal Resistance: The battery's internal components offer some resistance to the flow of electrons, leading to energy loss as heat. This internal resistance increases with age and use, impacting battery performance.
- Energy Storage Capacity: The amount of electrical energy a battery can store is determined by its chemical composition and physical size. Higher energy density batteries store more energy for the same size and weight.
The Future of Battery Technology and Energy Conversion
Research and development in battery technology are continuously pushing the boundaries of energy conversion efficiency and capacity. Areas of active research include:
- Solid-state batteries: Replacing liquid or gel electrolytes with solid electrolytes promises improved safety and higher energy density.
- Next-generation cathode materials: Exploring novel cathode materials with higher capacity and stability.
- Improved anode materials: Developing anodes that can store more lithium ions efficiently.
- Advanced battery management systems: Optimizing charging and discharging processes to maximize battery lifespan and safety.
Conclusion: A Powerful Engine of Modern Life
Batteries are fundamental to modern society, converting chemical energy into electrical energy to power our devices and increasingly, our transportation and energy grids. Understanding the underlying principles of this energy conversion – the electrochemical reactions, the different types of batteries, and the ongoing technological advancements – is crucial to appreciating their importance and fostering further innovation in this vital field. The ongoing quest for higher energy density, longer lifespan, improved safety, and sustainable materials will continue to shape the future of battery technology and its role in a more sustainable and electrified world.
Latest Posts
Latest Posts
-
The Element Present In Every Organic Compound Is
Apr 22, 2025
-
Which Of The Following Is A Correct Association
Apr 22, 2025
-
Equity Theory States That Employees Will Perform Well If They
Apr 22, 2025
-
As An Object Falls Freely In A Vacuum Its
Apr 22, 2025
-
How Many Protons Does Xe Have
Apr 22, 2025
Related Post
Thank you for visiting our website which covers about A Battery Converts What Type Of Energy To Another . We hope the information provided has been useful to you. Feel free to contact us if you have any questions or need further assistance. See you next time and don't miss to bookmark.